Analysis of baseline interstitial lung abnormality on the risk of checkpoint inhibitor-related pneumonitis and survival in advanced non-small cell lung cancer patients treated with first-line PD-1/PD-L1 inhibitors
Highlight box
Key findings
• Pre-existing interstitial lung abnormality (ILA) is found to be an independent risk factor that is strongly associated with checkpoint inhibitor-related pneumonitis (CIP), and is significantly associated with progression-free survival and overall survival (OS) in advanced non-small cell lung cancer (NSCLC) patients after first-line immunotherapy.
• Severe CIP is also associated with a shorter OS time.
What is known, and what is new?
• ILA is known to be strongly correlated with an increase in the risk of lung cancer, complications from cancer treatment, and mortality.
• Among NSCLC patients treated with first-line programmed cell death protein-1/programmed cell death-ligand 1 inhibitors, those with ILA are more likely to develop CIP and have a less favorable prognosis than those without ILA.
What is the implication, and what should change now?
• Our findings showed that closer clinical attention needs to be paid to ILA in the future.
• Further research needs to be conducted on pulmonary function tests and the specific imaging features of ILA on chest computed tomography.
Introduction
Lung cancer is the leading cause of cancer-related death (1). Programmed cell death protein-1 (PD-1) or programmed cell death-ligand 1 (PD-L1) monoclonal antibodies, as typical of immune checkpoint inhibitors (ICIs), have emerged as an increasingly recommended choice in the first-line treatment of non-small cell lung cancer (NSCLC) (2,3). However, ICIs have been documented to have significant negative consequences (4,5). As ICIs directly regulate the body’s immune function, immune-related adverse events (irAEs) can occur anywhere immune cells can reach (6). Checkpoint inhibitor-related pneumonitis (CIP) is a rare but potentially severe irAE (7).
Interstitial lung abnormalities (ILAs) include ground-glass or reticular abnormalities, traction bronchiectasis, honeycomb structures, and non-emphysematous cysts. ILAs that affect more than 5% of the lung area but have not yet been diagnosed or are clinically suspected of being interstitial lung disease (ILD) can occasionally be seen on chest computed tomography (CT) scans (8). ILAs have been found in approximately 2–10% of individuals who undergo screenings for lung cancer (9-11), and an even greater proportion of lung cancer patients exhibit ILAs (12). The percentage of ILA in lung cancer ranges from approximately 4–18% (13-17) and is slightly more common in Asian patients than in Caucasians in the available studies (12,14,17,18).
Zhang et al. have shown that immunotherapy is likely to cause CIP in lung cancer patients with ILD (19). Additionally, lung cancer patients with ILD have a worse prognosis than those without ILD (20,21). However, currently, there are no complete guidelines on how to diagnose and treat lung cancer patients with ILA. ILA has been shown to be strongly correlated with an increase in the risk of lung cancer, complications from cancer treatment, and mortality (12,18,22,23). A number of studies have shown that pre-existing ILA is closely associated with a high incidence of post-operative lung complications and reduced survival in early-stage NSCLC patients (14,24-26). Some studies have suggested that ILA may be a predictor of CIP in patients with NSCLC (27-29). However, as previous studies (28,30) have included all treatment lines of immunotherapy, the influence of other treatments on the occurrence of CIP cannot be ruled out. Research into the relationship between ILA and CIP risk and survival in advanced NSCLC patients undergoing first-line immunotherapy is very limited. Many studies have shown that specific imaging features on chest CT in patients with ILA are associated with prognosis (31,32). However, few studies have been conducted on NSCLC patients and ILA. Pulmonary function test (PFT) results could serve as potential risk factors in the development of CIP, but their role is not yet well understood (29,30,33). Thus, further studies need to be conducted on PFTs in advanced NSCLC patients.
To address the gaps in the existing research, we retrospectively examined the influence of ILAs and PFT results on the incidence of CIP and survival in advanced NSCLC patients treated with first-line PD-1/PD-L1 inhibitors. Additionally, we also examined the effects of specific imaging features on chest CT scans on patient outcomes. We present this article in accordance with the REMARK reporting checklist (available at https://tlcr.amegroups.com/article/view/10.21037/tlcr-2025-150/rc).
Methods
Study design and participants
This retrospective cohort study had a median follow-up time of 23.2 months (range, 19.8–26.6 months). We retrospectively studied advanced NSCLC patients after first-line PD-1/PD-L1 inhibitor therapy with or without other treatments at a single center, Jinling Hospital, between January 2018 and December 2023. The inclusion and exclusion criteria for the study are shown in Figure S1. All lung cancer patients were diagnosed by histological or cytological confirmation. Patients were excluded if they had not undergone a chest CT scan using our imaging system within 3 months before starting ICI therapy. Patients with a clinical diagnosis of ILD or idiopathic pulmonary fibrosis (IPF) were also excluded from the study. Patients with actionable genetic alterations (driver mutations) were equally excluded. The last follow-up of our study was 1 April 2024. Data were collected and managed using the hospital’s electronic medical record system. Patient data, including baseline demographics, clinical characteristics, treatment history, and laboratory tests, including age, smoking history, cancer type, Eastern Cooperative Oncology Group performance status (ECOG PS), history of chest radiation therapy, history of chronic obstructive pulmonary disease (COPD), history of gastroesophageal reflux disease (GERD), and the occurrence of CIP, were extracted from the electronic medical record system. We also collected specific imaging features on chest CT and PFT results.
Diagnosis of CIP
The American Society of Clinical Oncology (ASCO) guidelines were used to define and classify pneumonia. CIP was classified into four grades (7): grade 1: asymptomatic, limited to a single lobe or <25% of the lung parenchyma; grade 2: new/worsening symptoms involving multiple lobes and up to 25–50% of the lung parenchyma; grade 3: severe new symptoms involving all lobes or >50% of the lung parenchyma; and grade 4: life-threatening dyspnea, acute respiratory distress syndrome (ARDS), and so forth. Based on the previous study (34), we attributed the development of pneumonia to immunotherapy because of its association with the use of ICIs and the absence of other well-defined risk factors or etiologies, including infection. Two respiratory physicians recorded CIP cases at the onset of pneumonia, and the agreement between the two respiratory physicians’ opinions was assessed retrospectively.
Evaluation of pre-existing ILA
We reviewed the baseline high-resolution chest CT images of patients obtained before immunotherapy. On chest CT, abnormal images of up to 1 mm in diameter could be visualized. According to Fleischner’s guidelines (8), ILA was defined as an abnormality that affects more than 5% of any lung region and includes ground-glass or reticular abnormalities, architectural distortion, non-emphysematous cysts, traction bronchiectasis, and honeycomb structures. The guidelines further subdivide ILA into non-subpleural, subpleural non-fibrotic, and subpleural fibrotic-ILA (SF-ILA) (8). Fibrotic ILA refers to the presence of traction bronchiectasis and/or honeycomb structures (35-37).
Two experienced respiratory physicians assessed and graded the severity of ILA. The ILAs were classified into four grades ranging from 0 to 3 (38); a score of 0 indicated no ILA; a score of 1 indicated focal or unilateral ground-glass opacities (GGOs), focal or unilateral reticular structures, or patchy GGOs (affecting less than 5% of the lung); a score of 2 indicated an abnormality affecting more than 5% of any lung region, and included ground-glass or reticular abnormalities, architectural distortion, non-emphysematous cysts, traction bronchiectasis, and honeycomb structures; a score of 3 indicated bilateral multilobe fibrosis with the subpleural distribution of honeycomb structures or traction bronchiectasis. If any disagreements arose between the two respiratory physicians, the final score was determined by consensus. In addition, we have involved radiologists in the diagnosis of ILA for controversial areas. Cohen’s kappa was used to assess the agreement between the scores of the two respiratory physicians.
Evaluation of PFT results
Prior to the inhalation of bronchodilators, we recorded the baseline PFT results of the patients, including forced vital capacity (FVC), forced expiratory volume in 1 s (FEV1), the FEV1/FVC ratio, maximal expiratory flow (MEF), peak expiratory flow (PEF), maximal voluntary ventilation (MVV), maximum mid-expiratory flow (MMEF), expiratory vital capacity (VC EX), inspiratory vital capacity (VC IN), and maximum lung capacity (VC MAX). The PFT results closest to the start of immunotherapy were selected for the analysis. All values were recorded as ratios or predicted percentages adjusted for the patient’s height, weight, sex, and age.
Statistical analysis
The continuous variables are expressed as the mean ± standard deviation, and median (interquartile), and the categorical variables are expressed as the frequency (percentage). The t-test was used for the continuous variables with a normal distribution, and the Wilcoxon test was used for those with a non-normal distribution. For the categorical variables, the Pearson Chi-squared test was used to compare the differences between the two groups.
Risk factors for CIP were identified by logistic regression analysis. Cox proportional hazards models were used to examine the significance of ILA and specific imaging features of ILA on chest CT in predicting progression-free survival (PFS) and overall survival (OS). Variables that were significant (P<0.05) in the univariate analysis were included in the multivariate analysis. Both PFS and OS were estimated using the Kaplan-Meier method.
SPSS version 27.0 (IBM Inc., Chicago, IL, USA) and GraphPad Prism 10.0 (GraphPad, San Diego, CA, USA) were used to perform all the statistical analyses. A P value <0.05 was considered statistically significant. Receiver operating characteristic curves were used to determine the cut-off values for the continuous variables.
Ethical statement
This study was conducted in accordance with the Declaration of Helsinki (as revised in 2013). It was approved by the Ethics Committee of the Jinling Hospital (No. 2024DZKY-051-01). As this was a retrospective and observational study, the requirement of informed consent was waived. Patient information was kept confidential.
Results
Baseline characteristics
In total, 269 patients with advanced NSCLC were enrolled in the study, of whom 93 (34.57%) had ILA, and 176 (65.43%) did not have ILA. Among the patients without ILA, 92 (52.27%) had a score of 0, and 84 (47.73%) had a score of 1. Among the patients with ILA, 86 (92.47%) had a score of 2, and 7 (7.53%) had a score of 3 (Figure S1). A total of 39 (14.50%) patients developed CIP, of whom 13 (33.33%) had grade 1, 12 (30.77%) had grade 2, 11 (28.21%) had grade 3, and 3 (7.69%) had grade 4.
Table 1 presents the baseline demographics and characteristics of the 269 patients, of whom 223 (82.90%) were male, and 122 (45.35%) were under 65 years old. A total of 185 patients (68.77%) had a history of smoking, and 234 (86.99%) had an ECOG PS score of 0–1. Additionally, 136 patients (50.56%) had squamous cell carcinoma, and 124 (46.10%) had adenocarcinoma. In relation to the tumor-node-metastasis (TNM)-based staging, 122 patients (45.35%) were diagnosed with stage III lung cancer, and 147 patients (54.65%) were diagnosed with stage IV. Additionally, 39 patients (14.50%) had COPD at the baseline, and 57 (21.19%) had previously received chest radiation therapy. Of all first-line treatments, 76 patients (28.25%) received ICI monotherapy and 193 (71.75%) received ICI combination therapy. Two hundred and fifty patients (92.94%) were treated with first-line PD-1 inhibitors, mainly including sintilimab/camrelizumab/tislelizumab/toripalimab/serplulimab/pembrolizumab/nivolumab/penpulimab. Nineteen patients (7.06%) were treated with first-line PD-L1 inhibitors, mainly including sugemalimab/atezolizumab/durvalumab/envafolimab. Table 1 also shows the laboratory examination results of the patients at the baseline. Figure S1 shows the flowchart of our study.
Table 1
Variables | Total | Group 1 (without CIP) | Group 2 (with CIP) | P value |
---|---|---|---|---|
Patient | 269 | 230 | 39 | – |
Age (years) | 0.35 | |||
<65 | 122 (45.35) | 107 (46.52) | 15 (38.46) | |
≥65 | 147 (54.65) | 123 (53.48) | 24 (61.54) | |
Sex | 0.44 | |||
Male | 223 (82.90) | 189 (82.17) | 34 (87.18) | |
Female | 46 (17.10) | 41 (17.83) | 5 (12.82) | |
Smoking history | 0.66 | |||
Never | 84 (31.23) | 73 (31.74) | 11 (28.21) | |
Former/current | 185 (68.77) | 157 (68.26) | 28 (71.79) | |
ECOG PS | 0.63 | |||
0–1 | 234 (86.99) | 201 (87.39) | 33 (84.62) | |
≥2 | 35 (13.01) | 29 (12.61) | 6 (15.38) | |
BMI (kg/m2) | 0.005 | |||
<24.12 | 183 (68.03) | 164 (71.30) | 19 (48.72) | |
≥24.12 | 86 (31.97) | 66 (28.70) | 20 (51.28) | |
Co-morbidities | ||||
GERD | 3 (1.12) | 2 (0.87) | 1 (2.56) | 0.35 |
COPD | 39 (14.50) | 32 (13.91) | 7 (17.95) | 0.51 |
Histology | 0.71 | |||
LUSC | 136 (50.56) | 118 (51.30) | 18 (46.15) | |
LUAD | 124 (46.10) | 105 (45.65) | 19 (48.72) | |
Other NSCLC | 9 (3.35) | 7 (3.04) | 2 (5.13) | |
Clinical stage | 0.42 | |||
III | 122 (45.35) | 102 (44.35) | 20 (51.28) | |
IV | 147 (54.65) | 128 (55.65) | 19 (48.72) | |
Treatment regimen | 0.70 | |||
ICI monotherapy | 76 (28.25) | 66 (28.70) | 10 (25.64) | |
ICI combination therapy | 193 (71.75) | 164 (71.30) | 29 (74.36) | |
ICI type | 0.13 | |||
PD-1 | 250 (92.94) | 216 (93.91) | 34 (87.18) | |
Sintilimab | 87 (32.34) | 74 (32.17) | 13 (33.33) | |
Camrelizumab | 60 (22.30) | 51 (22.17) | 9 (23.08) | |
Tislelizumab | 64 (23.79) | 54 (23.48) | 10 (25.64) | |
Toripalimab | 9 (3.35) | 9 (3.91) | 0 (0.00) | |
Serplulimab | 12 (4.46) | 11 (4.78) | 1 (2.56) | |
Pembrolizumab | 15 (5.58) | 14 (6.09) | 1 (2.56) | |
Nivolumab | 2 (0.74) | 2 (0.87) | 0 (0.00) | |
Penpulimab | 1 (0.37) | 1 (0.43) | 0 (0.00) | |
PD-L1 | 19 (7.06) | 14 (6.09) | 5 (12.82) | |
Sugemalimab | 15 (5.58) | 12 (5.22) | 3 (7.69) | |
Atezolizumab | 2 (0.74) | 1 (0.43) | 1 (2.56) | |
Durvalumab | 1 (0.37) | 0 (0.00) | 1 (2.56) | |
Envafolimab | 1 (0.37) | 1 (0.43) | 0 (0.00) | |
PD-L1 tumor proportion score | 0.26 | |||
≥50 | 16 (5.95) | 16 (6.96) | 0 (0.00) | |
1–49 | 29 (10.78) | 24 (10.43) | 5 (12.82) | |
<1 | 39 (14.50) | 31 (13.48) | 8 (20.51) | |
Not tested | 185 (68.77) | 159 (69.13) | 26 (66.67) | |
Prior chest radiation therapy | 57 (21.19) | 51 (22.17) | 6 (15.38) | 0.34 |
Laboratory examination | ||||
Cyfra21-1 (ng/mL) | 0.01 | |||
<4.02 | 67 (24.91) | 51 (22.17) | 16 (41.03) | |
≥4.02 | 202 (75.09) | 179 (77.83) | 23 (58.97) | |
NSE (μg/L) | 0.18 | |||
<11.81 | 79 (29.37) | 64 (27.83) | 15 (38.46) | |
≥11.81 | 190 (70.63) | 166 (72.17) | 24 (61.54) | |
CEA (μg/L) | 0.048 | |||
<2.10 | 69 (25.65) | 54 (23.48) | 15 (38.46) | |
≥2.10 | 200 (74.35) | 176 (76.52) | 24 (61.54) | |
CA125 (U/mL) | 0.43 | |||
<57.35 | 150 (55.76) | 126 (54.78) | 24 (61.54) | |
≥57.35 | 119 (44.24) | 104 (45.22) | 15 (38.46) | |
FIB (g/L) | 0.07 | |||
<4.78 | 188 (69.89) | 156 (67.83) | 32 (82.05) | |
≥4.78 | 81 (30.11) | 74 (32.17) | 7 (17.95) | |
ALB (g/L) | 0.03 | |||
<37.05 | 147 (54.65) | 132 (57.39) | 15 (38.46) | |
≥37.05 | 122 (45.35) | 98 (42.61) | 24 (61.54) | |
IL-6 (ng/L) | 0.09 | |||
<17.73 | 105 (39.03) | 85 (36.96) | 20 (51.28) | |
≥17.73 | 164 (60.97) | 145 (63.04) | 19 (48.72) | |
LDH (U/L) | 0.04 | |||
<186.50 | 110 (40.89) | 100 (43.48) | 10 (25.64) | |
≥186.50 | 159 (59.11) | 130 (56.52) | 29 (74.36) | |
NE (×109/L) | 0.10 | |||
<3.29 | 68 (25.28) | 54 (23.48) | 14 (35.90) | |
≥3.29 | 201 (74.72) | 176 (76.52) | 25 (64.10) | |
Lym (×109/L) | 0.26 | |||
<1.17 | 70 (26.02) | 57 (24.78) | 13 (33.33) | |
≥1.17 | 199 (73.98) | 173 (75.22) | 26 (66.67) | |
Mono (×109/L) | 0.33 | |||
<0.41 | 80 (29.74) | 71 (30.87) | 9 (23.08) | |
≥0.41 | 189 (70.26) | 159 (69.13) | 30 (76.92) | |
Eos (×109/L) | 0.056 | |||
<0.22 | 199 (73.98) | 175 (76.09) | 24 (61.54) | |
≥0.22 | 70 (26.02) | 55 (23.91) | 15 (38.46) | |
NLR | 0.07 | |||
<3.57 | 180 (66.91) | 149 (64.78) | 31 (79.49) | |
≥3.57 | 89 (33.09) | 81 (35.22) | 8 (20.51) | |
MLR | 0.20 | |||
<0.35 | 147 (54.65) | 122 (53.04) | 25 (64.10) | |
≥0.35 | 122 (45.35) | 108 (46.96) | 14 (35.90) | |
Autoantibody | 0.94 | |||
Positive | 91 (33.83) | 78 (33.91) | 13 (33.33) | |
Not tested or negative | 178 (66.17) | 152 (66.09) | 26 (66.67) |
Data are presented as n or n (%). ALB, albumin; BMI, body mass index; CA125, carbohydrate antigen 125; CEA, carcinoembryonic antigen; CIP, checkpoint inhibitor-related pneumonitis; COPD, chronic obstructive pulmonary disease; Cyfra21-1, cytokeratin 19 fragment antigen 21-1; ECOG PS, Eastern Cooperative Oncology Group performance status; Eos, eosinophil; FIB, fibrinogen; GERD, gastroesophageal reflux disease; ICI, immune checkpoint inhibitor; IL-6, interleukin-6; LDH, lactate dehydrogenase; LUSC, lung squamous cell carcinoma; LUAD, lung adenocarcinoma; Lym, lymphocyte; MLR, monocyte-to-lymphocyte ratio; Mono, monocyte; NE, neutrophil; NLR, neutrophil-to-lymphocyte ratio; NSE, neuron-specific enolase; NSCLC, non-small cell lung cancer; PD-1, programmed cell death protein-1; PD-L1, programmed cell death-ligand 1.
Pre-existing ILA on chest CT
The 269 patients were divided into two groups: the with ILA group, and the without ILA group. Table S1 sets out the specific baseline characteristics of the patients, and the univariate analysis results. Representative CT images of ILA on chest CT scans are shown in Figure 1. Figure 1G showed the progression of ILA. A 68-year-old man was diagnosed with squamous cell carcinoma stage IV. After five cycles of chemotherapy combined with sugemalimab immunotherapy, the left lung subpleural ground-glass shadow was more absorbed than before, and a right lung subpleural reticular shadow was newly developed. CIP development. In Figure 1H, we displayed the development of CIP. A 58-year-old man was diagnosed with squamous cell carcinoma stage IV. After four cycles of chemotherapy combined with penpulimab immunotherapy, the patient developed grade 3 CIP, immunotherapy was discontinued and prednisone treatment was administered. Of the 269 patients, 177 had ground-glass attenuation (65.80%), 50 had reticular shadows (18.59%), 11 had honeycomb structures (4.09%), 8 had traction bronchiectasis (2.97%), and 5 had non-emphysematous cysts (1.86%) on chest CT. Among the ILA patients, 32 (34.41%) had the non-subpleural subtype, 48 (51.61%) had the subpleural nonfibrotic subtype, and 13 (13.98%) had the subpleural fibrotic subtype (Table 2).
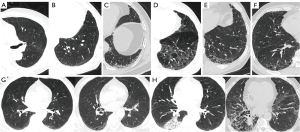
Table 2
Variables | Total | Group 1 (without CIP) | Group 2 (with CIP) |
---|---|---|---|
Patient | 269 | 230 | 39 |
Score of ILA | |||
0 | 92 (34.20) | 84 (36.52) | 8 (20.51) |
1 | 84 (31.23) | 77 (33.48) | 7 (17.95) |
2 | 86 (31.97) | 63 (27.39) | 23 (58.97) |
3 | 7 (2.60) | 6 (2.61) | 1 (2.56) |
ILA | |||
ILA+ | 93 (34.57) | 69 (30.00) | 24 (61.54) |
ILA− | 176 (65.43) | 161 (70.00) | 15 (38.46) |
Subcategories of ILA | |||
Non-subpleural | 32 (11.90) | 21 (9.13) | 11 (28.21) |
Subpleural nonfibrotic | 48 (17.84) | 37 (16.09) | 11 (28.21) |
Subpleural fibrotic | 13 (4.83) | 11 (4.78) | 2 (5.13) |
Ground-glass attenuation | |||
Negative | 92 (34.20) | 84 (36.52) | 8 (20.51) |
Positive | 177 (65.80) | 146 (63.48) | 31 (79.49) |
Reticular shadow | |||
Negative | 219 (81.41) | 188 (81.74) | 31 (79.49) |
Positive | 50 (18.59) | 42 (18.26) | 8 (20.51) |
Honeycomb structure | |||
Negative | 258 (95.91) | 221 (96.09) | 37 (94.87) |
Positive | 11 (4.09) | 9 (3.91) | 2 (5.13) |
Traction bronchiectasis | |||
Negative | 261 (97.03) | 224 (97.39) | 37 (94.87) |
Positive | 8 (2.97) | 6 (2.61) | 2 (5.13) |
Non-emphysematous cysts | |||
Negative | 264 (98.14) | 226 (98.26) | 38 (97.44) |
Positive | 5 (1.86) | 4 (1.74) | 1 (2.56) |
Data are presented as n or n (%). CIP, checkpoint inhibitor-related pneumonitis; CT, computed tomography; ILA, interstitial lung abnormality.
Risk factors for CIP
In the 39 patients with CIP in our study, the time to onset of CIP ranged from 10 days to 30 months after receiving first-line immunotherapy, with a median time to onset of about 4 months.
Baseline data and univariate analysis by CIP status in patients with ILA are shown in Table S2. Table 2 details the distribution of ILA and specific imaging features based on the occurrence of CIP. Univariate and multivariate logistic regression analyses were performed to assess the association between ILA and CIP (Table 3). Pre-existing ILA [odds ratio (OR): 3.733; 95% confidence interval (CI): 1.846–7.549; P<0.001], body mass index (BMI) (≥24.12 kg/m2) (OR: 2.616; 95% CI: 1.312–5.214; P=0.006), and lactate dehydrogenase (LDH) (≥186.50 U/L) (OR: 2.231; 95% CI: 1.038–4.792; P=0.04) were found to be significantly associated with CIP. The multivariate analysis showed that ILA (OR: 4.128; 95% CI: 1.984–8.587; P<0.001) and BMI (≥24.12 kg/m2) (OR: 2.955; 95% CI: 1.423–6.139; P=0.004) were robust independent predictors of CIP.
Table 3
Variables | Univariate analysis | Multivariate analysis | |||
---|---|---|---|---|---|
OR (95% CI) | P value | OR (95% CI) | P value | ||
Age (≥65 years) | 1.392 (0.695, 2.789) | 0.35 | |||
Female | 0.678 (0.250, 1.838) | 0.45 | |||
Former/current smoking | 1.184 (0.559, 2.507) | 0.66 | |||
ECOG PS (≥2) | 1.260 (0.486, 3.268) | 0.63 | |||
BMI (≥24.12 kg/m2) | 2.616 (1.312, 5.214) | 0.006 | 2.955 (1.423, 6.139) | 0.004 | |
COPD | 1.354 (0.551, 3.326) | 0.51 | |||
GERD | 3.000 (0.265, 33.903) | 0.38 | |||
Prior chest radiation therapy | 0.638 (0.253, 1.607) | 0.34 | |||
ILA | 3.733 (1.846, 7.549) | <0.001 | 4.128 (1.984, 8.587) | <0.001 | |
NSE (≥11.81 μg/L) | 0.617 (0.304, 1.250) | 0.18 | |||
CEA (≥2.10 μg/L) | 0.491 (0.241, 1.002) | 0.051 | |||
CA125 (≥57.35 U/mL) | 0.757 (0.378, 1.518) | 0.43 | |||
FIB (≥4.78 g/L) | 0.461 (0.194, 1.093) | 0.08 | |||
IL-6 (≥17.73 ng/L) | 0.557 (0.281, 1.102) | 0.09 | |||
LDH (≥186.50 U/L) | 2.231 (1.038, 4.792) | 0.04 | 2.195 (0.991, 4.859) | 0.053 | |
NE (≥3.29×109/L) | 0.548 (0.266, 1.128) | 0.10 | |||
Lym (≥1.17×109/L) | 0.659 (0.318, 1.367) | 0.26 | |||
Mono (≥0.41×109/L) | 1.488 (0.672, 3.298) | 0.33 | |||
Eos (≥0.22×109/L) | 1.989 (0.975, 4.056) | 0.059 | |||
NLR (≥3.57) | 0.475 (0.208, 1.081) | 0.08 | |||
MLR (≥0.35) | 0.633 (0.313, 1.279) | 0.20 | |||
Positive autoantibody | 0.974 (0.474, 2.001) | 0.94 |
BMI, body mass index; CA125, carbohydrate antigen 125; CEA, carcinoembryonic antigen; CI, confidence interval; CIP, checkpoint inhibitor-related pneumonitis; COPD, chronic obstructive pulmonary disease; ECOG PS, Eastern Cooperative Oncology Group performance status; Eos, eosinophil; FIB, fibrinogen; GERD, gastroesophageal reflux disease; ILA, interstitial lung abnormality; IL-6, interleukin-6; LDH, lactate dehydrogenase; Lym, lymphocyte; MLR, monocyte-to-lymphocyte ratio; Mono, monocyte; NE, neutrophil; NLR, neutrophil-to-lymphocyte ratio; NSE, neuron-specific enolase; OR, odds ratio.
No association between specific imaging features on chest CT and CIP in all patients was found. In the patients with ILA, no statistically significant correlation between the subtypes of ILA and CIP was found (Table S3).
Of the patients with CIP, 25 (64.10%) had mild CIP (grades 1/2), and 14 (35.90%) had severe CIP (grades 3/4). OS did not differ significantly between the patients with CIP and those without CIP (median for patients with CIP: 28.9 months; median for patients without CIP: 35 months) [with CIP vs. without CIP: hazard ratio (HR): 1.588; 95% CI: 0.907–2.781; P=0.11] (Figure 2A). Compared to the patients with mild CIP (grades 1/2), those with severe CIP (grades 3/4) had worse OS (severe CIP vs. mild CIP: median for patients with grade 3/4: 12.4 months; median for patients with grade 1/2: 35.8 months) (HR: 4.808; 95% CI: 1.671–13.830; P=0.004) (Figure 2B). Furthermore, in patients with ILA, we did not find a correlation between PFS (P=0.33) and OS (P=0.63) in patients who developed CIP or not (Figure S2).
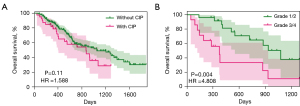
Survival analysis
As Figure 3A shows, the median PFS of the patients with ILA was 7.8 months, while that of the patients without ILA was 18.7 months (ILA+vs. ILA−: HR: 1.965; 95% CI: 1.373–2.812; P<0.001). ILA was also associated with a shorter OS time; the patients with ILA had a median OS of 21.1 months, while those without ILA had a median OS of 42.5 months (ILA+vs. ILA−: HR: 2.213; 95% CI: 1.404–3.488; P<0.001) (Figure 3B).
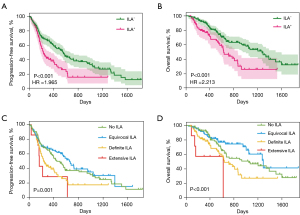
Table 4 shows the univariate Cox regression analysis results in terms of PFS and OS. Variables that were significant in predicting PFS and OS in the univariate analysis were included in the multivariate analysis.
Table 4
Variables | PFS | OS | |||
---|---|---|---|---|---|
HR (95% CI) | P value | HR (95% CI) | P value | ||
(A) | |||||
Age (≥65 years) | 1.090 (0.799, 1.485) | 0.59 | 1.036 (0.704, 1.524) | 0.86 | |
Female | 1.139 (0.774, 1.676) | 0.51 | 0.739 (0.439, 1.244) | 0.25 | |
Former/current smoking | 0.777 (0.564,1.070) | 0.12 | 1.055 (0.693, 1.605) | 0.80 | |
ECOG PS (≥2) | 1.260 (0.796, 1.995) | 0.33 | 1.474 (0.835, 2.604) | 0.18 | |
COPD | 1.209 (0.769, 1.901) | 0.41 | 1.304 (0.739, 2.298) | 0.36 | |
GERD | 1.406 (0.348, 5.679) | 0.63 | 1.480 (0.205, 10.670) | 0.70 | |
ILA | 1.826 (1.320, 2.525) | <0.001 | 1.996 (1.334, 2.988) | <0.001 | |
Cyfra21-1 (≥7.40 ng/mL) | 1.671 (1.224, 2.282) | 0.001 | 1.882 (1.246, 2.842) | 0.003 | |
NSE (≥12.90 μg/L) | 1.177 (0.863, 1.606) | 0.30 | 1.370 (0.918, 2.046) | 0.12 | |
CEA (≥131.34 μg/L) | 2.442 (1.510, 3.950) | <0.001 | 2.807 (1.666, 4.730) | <0.001 | |
CA125 (≥96.35 U/mL) | 1.918 (1.344, 2.737) | <0.001 | 1.661 (1.076, 2.562) | 0.02 | |
FIB (≥4.31 g/L) | 1.177 (0.870, 1.592) | 0.29 | 1.898 (1.282, 2.810) | 0.001 | |
IL-6 (≥14.86 ng/L) | 1.082 (0.785, 1.490) | 0.63 | 1.915 (1.214, 3.022) | 0.005 | |
LDH (≥169.50 U/L) | 1.220 (0.862, 1.725) | 0.26 | 2.012 (1.223, 3.309) | 0.006 | |
ALB (≥33.30 g/L) | 0.754 (0.523, 1.086) | 0.13 | 0.644 (0.409, 1.014) | 0.057 | |
NE (≥6.19×109/L) | 1.547 (1.079, 2.218) | 0.02 | 1.536 (0.986, 2.394) | 0.058 | |
Lym (≥1.26×109/L) | 1.035 (0.744, 1.442) | 0.84 | 1.395 (0.889, 2.188) | 0.15 | |
Mono (≥0.39×109/L) | 1.246 (0.868, 1.790) | 0.23 | 2.014 (1.182, 3.430) | 0.01 | |
Eos (≥0.32×109/L) | 0.778 (0.482, 1.255) | 0.30 | 0.633 (0.319, 1.254) | 0.19 | |
NLR (≥2.63) | 1.192 (0.874, 1.626) | 0.27 | 1.520 (1.013, 2.282) | 0.043 | |
MLR (≥0.29) | 1.217 (0.894, 1.658) | 0.21 | 1.759 (1.168, 2.649) | 0.007 | |
Positive autoantibody | 1.085 (0.787, 1.496) | 0.62 | 1.007 (0.666, 1.524) | 0.97 | |
(B) | |||||
Ground-glass attenuation | 1.156 (0.842, 1.588) | 0.37 | 1.107 (0.744, 1.648) | 0.62 | |
Reticular shadow | 1.557 (1.083, 2.237) | 0.02 | 1.704 (1.080, 2.690) | 0.02 | |
Honeycomb structure | 1.579 (0.775, 3.216) | 0.21 | 2.162 (0.877, 5.331) | 0.09 | |
Traction bronchiectasis | 0.790 (0.292, 2.133) | 0.64 | 0.600 (0.148, 2.438) | 0.48 | |
Non-emphysematous cysts | 2.551 (0.942, 6.911) | 0.07 | 2.971 (0.932, 9.469) | 0.07 | |
(C) | |||||
Non-subpleural | 0.853 (0.496, 1.468) | 0.57 | 1.351 (0.715, 2.553) | 0.36 | |
Subpleural nonfibrotic | 0.998 (0.604, 1.649) | 0.99 | 0.701 (0.376, 1.310) | 0.27 | |
Subpleural fibrotic | 1.332 (0.675, 2.627) | 0.41 | 1.174 (0.490, 2.810) | 0.72 |
(A) Univariate analysis based on baseline characteristics and laboratory examination results. (B) Univariate analysis based on specific imaging features. (C) Univariate analysis based on subcategories of ILAs. ALB, albumin; CA125, carbohydrate antigen 125; CEA, carcinoembryonic antigen; CI, confidence interval; COPD, chronic obstructive pulmonary disease; Cyfra21-1, cytokeratin 19 fragment antigen 21-1; ECOG PS, Eastern Cooperative Oncology Group performance status; Eos, eosinophil; FIB, fibrinogen; GERD, gastroesophageal reflux disease; HR, hazard ratio; ILA, interstitial lung abnormality; IL-6, interleukin-6; LDH, lactate dehydrogenase; Lym, lymphocyte; MLR, monocyte-to-lymphocyte ratio; Mono, monocyte; NE, neutrophil; NLR, neutrophil-to-lymphocyte ratio; NSE, neuron-specific enolase; OS, overall survival; PFS, progression-free survival.
After the multivariate analysis, pre-existing ILA (HR: 1.621; 95% CI: 1.165–2.255; P=0.004), cytokeratin 19 fragment antigen 21-1 (Cyfra21-1) (≥7.40 ng/mL) (HR: 1.462; 95% CI: 1.063–2.013; P=0.02), carcinoembryonic antigen (CEA) (≥131.34 µg/L) (HR: 1.936; 95% CI: 1.130–3.315; P=0.02), and neutrophil (NE) (≥6.19×109/L) (HR: 1.468; 95% CI: 1.011–2.130; P=0.044) were found to be significantly associated with worse PFS (Table S4).
The outcomes of the multivariable Cox regression analysis for OS are shown in Table S5. Of the possible risk factors, the presence of ILA (HR: 1.899; 95% CI: 1.253–2.878; P=0.002), CEA (≥131.34 µg/L) (HR: 3.187; 95% CI: 1.787–5.687; P<0.001), interleukin-6 (IL-6) (≥14.86 ng/L) (HR: 1.707; 95% CI: 1.047–2.783; P=0.03), and LDH (≥169.50 U/L) (HR: 2.141; 95% CI: 1.270–3.609; P=0.004) were found to be independently associated with OS. A reticular shadow on chest CT predicted a shorter PFS time (HR: 1.557; 95% CI: 1.083–2.237; P=0.02) and OS time (HR: 1.704; 95% CI: 1.080–2.690; P=0.02) (Table 4). ILA subtype was not found to have a significant effect on PFS and OS (Table 4). The patients with higher ILA scores had a significantly shorter PFS time (P=0.001) and OS time (P<0.001) than those with lower ILA scores (Figure 3C,3D). However, no ILA is worse in terms of outcome than equivocal ILA in Figure 3C,3D.
Pretreatment PFT results
Of the 269 patients treated with ICIs, 210 (78.07%) had PFT results before commencing ICI therapy. We found that PFT results before commencing ICI therapy were not statistically significant in predicting the occurrence of CIP (Table 5).
Table 5
Variables | Total (n=210) | Group 1 (without CIP) (n=178) | Group 2 (with CIP) (n=32) | P value |
---|---|---|---|---|
VC IN (% predicted) | 77.06±17.01 | 77.10±17.17 | 76.76±16.30 | 0.29 |
VC EX (% predicted) | 81.67±17.77 | 81.68±17.93 | 81.60±17.02 | 0.25 |
VC MAX (% predicted) | 82.18±17.60 | 82.16±17.74 | 82.28±17.00 | 0.94 |
FVC (% predicted) | 84.60±18.56 | 84.68±18.66 | 84.00±18.30 | 0.80 |
<80% | 90 (42.86) | 77 (43.26) | 13 (40.63) | 0.78 |
≥80% | 120 (57.14) | 101 (56.74) | 19 (59.38) | |
FEV1 (% predicted) | 72.61±20.43 | 72.57±20.58 | 72.88±19.83 | 0.80 |
<80% | 132 (62.86) | 112 (62.92) | 20 (62.50) | 0.96 |
≥80% | 78 (37.14) | 66 (37.08) | 12 (37.50) | |
FEV1/FVC (%) | 68.24 (59.70, 77.10) | 68.78 (59.77, 77.08) | 65.92 (59.33, 78.10) | 0.51 |
PEF (% predicted) | 59.71±22.49 | 59.32±22.41 | 62.30±23.39 | 0.20 |
MEF 50 (% predicted) | 41.80 (26.20, 63.70) | 41.95 (27.50, 64.93) | 39.00 (22.80, 58.90) | 0.88 |
MEF 25 (% predicted) | 39.25 (25.53, 55.50) | 40.85 (24.88, 55.65) | 35.50 (25.80, 54.58) | 0.90 |
MMEF 75/25 (%) | 1.20 (0.81, 1.92) | 1.22 (0.89, 1.90) | 0.97 (0.69, 2.08) | 0.72 |
MVV (% predicted) | 57.94 (43.65, 69.73) | 57.94 (43.43, 69.63) | 57.94 (52.03, 71.45) | 0.33 |
Data are presented as mean ± SD, n (%), or median (interquartile). CIP, checkpoint inhibitor-related pneumonitis; FEV1, forced expiratory volume in 1 s; FVC, forced vital capacity; MEF, maximal expiratory flow; MMEF, maximum mid-expiratory flow; MVV, maximal voluntary ventilation; PEF, peak expiratory flow; PFT, pulmonary function test; SD, standard deviation; VC EX, expiratory vital capacity; VC IN, inspiratory vital capacity; VC MAX, maximum lung capacity.
Discussion
In this single-center retrospective cohort study, we found that of the advanced NSCLC patients who received PD-1/PD-L1 inhibitor therapy, those with pre-existing ILA had a substantially increased risk of CIP, and shorter PFS and OS times. This was the first study to simultaneously examine the effect of ILA on CIP and survival in patients with advanced NSCLC. This study was also the first to focus exclusively on first-line immunotherapy, thereby eliminating confounding by other lines of treatment. Our findings revealed that patients with pre-existing ILA may be a potentially vulnerable group. Therefore, lung cancer patients with ILA need to be closely observed and monitored. Prior to immunotherapy, radiological features on chest CT can be used to assess a patient’s risk of developing CIP and survival.
Based on previous studies, there has been controversy as to whether the existence of ILA is predictive of CIP. In a retrospective analysis (38), Horiuchi et al. did not find any association between ILA and CIP, or any association between the radiological features of ILA and CIP. However, many studies have reported an association between ILA and CIP (28-30). In the present study, ILA (P<0.001) was found to be significantly highly correlated with CIP after excluding confounding factors. The potential reasons for this result may be that patients with pre-existing ILA may have a more severe immune response after immunotherapy, that immune status and immune system function play an important role in many patients with ILD, and that there is evidence that the immune response is associated with progressive lung inflammation and interstitial fibrosis (39). It has been shown that Bronchoalveolar lavage lymphocytosis is a hallmark of immunity for the development of CIP, and that CIP is associated with upregulation of IL-1βhi monocytes in IL-1RA-expressing B cells. Immunotherapy may induce a similar mechanism leading to an increase in Bronchoalveolar lavage lymphocytes (mainly CD4+ T cells), resulting in an excessive inflammatory response leading to the development of CIP (40).
We also found that BMI (≥24.12 kg/m2) (P=0.004) may also be an influential factor in the development of CIP. A previous study showed that an increase in BMI after neoadjuvant immunochemotherapy is a risk factor for the development of CIP in NSCLC patients (41). A study has shown that patients with a BMI >25 kg/m2 are at increased risk of developing high-grade irAEs (42). We suspect that the inflammatory factors released by adipose tissue in obese patients may also be involved in the development of CIP (40). Obese adipose tissue secretes several types of pro-inflammatory adipokines, such as monocyte chemotactic protein-1, tumor necrosis factor-α, IL-1β, and IL-6 (43). In univariate analyses, we did not find a statistically significant association between IL-6 and the occurrence of CIP (OR: 0.557; P=0.09). Further studies are needed to confirm the role of adipose tissue in patients with CIP.
Chu et al. reported that the higher the peripheral blood eosinophil count, the greater the chance of developing CIP (44). In the present study, we found no significant correlation between the eosinophilic count (P=0.059) and the occurrence of CIP. However, the reasons for the differences in these results may be related to specific populations, and further prospective trials need to be conducted to examine this issue in the future.
Studies have (15,16) found that pre-existing ILA was linked to a shorter OS time in advanced lung cancer patients under both chemotherapy and targeted therapy. In our study, in advanced NSCLC patients after PD-1/PD-L1 inhibitor therapy, the detection of ILA on primary chest CT scanning was significantly correlated with worse PFS (P=0.004) and OS (P=0.002). We also found that some laboratory test markers may be essential factors affecting PFS and OS. To date, no other studies have examined how laboratory test markers affect PFS and OS in patients with advanced NSCLC. Therefore, our findings regarding these markers may be clinically significant. In our study, the patients with severe pneumonitis (grade 3/4) had a shorter OS time than those with mild pneumonitis (grade 1/2) (P=0.004). We speculate that acute exacerbation of interstitial pneumonia and a high incidence of CIP may explain the shortened OS in the baseline ILA patients. Lin et al. show that patients with mild irAEs have a better prognosis compared to those without irAEs. Subgroup analyses of irAE types showed that irAEs (thyroid dysfunction and gastrointestinal, cutaneous or endocrine irAEs) were associated with better PFS and OS. However, no significant differences were observed between patients with pneumonia or hepatobiliary irAEs (45). No ILA is worse in terms of PFS and OS in contrast to equivocal ILA in our study. The poorer prognosis of patients with lung cancer without ILA may be due to a combination of more aggressive molecular subtypes, suppression of the immune microenvironment, therapeutic resistance and diagnostic delay. In contrast, patients with combined ambiguous ILA may benefit from the immunosurveillance effects of chronic inflammation, earlier tumor detection and sensitivity of specific molecular features to therapy. Future validation of these hypotheses through multi-omics studies (e.g., genomic, immune-microenvironmental analyses) and prospective cohorts is needed to optimize individualized treatment strategies. The association between ILA and lung cancer mortality risk is complex and multifactorial, involving synergistic effects of chronic inflammation, fibrotic microenvironment, immune dysregulation, genetic susceptibility, and environmental exposures. In the future, longitudinal cohort studies and molecular mechanism exploration are needed to identify the key pathways to develop targeted intervention strategies (e.g., anti-inflammatory, anti-fibrotic combined with immunotherapy) (39,40,46,47).
The influence of specific imaging features on chest CT and subcategories of ILA on CIP and survival in advanced NSCLC patients are inconclusive. In our study, we did not find an association between specific imaging features on chest CT and CIP; however, previous findings differ (28,29). Additionally, we did not find that the subcategories of ILA affected the occurrence of CIP, which is consistent with the findings of Petranovic et al. (28). Kikuchi et al. found that SF-ILAs independently predicted CIP (OR: 5.35; 95% CI: 1.62–17.61; P=0.006) (48). SF-ILAs are strong independent predictors of CIP development in NSCLC patients and may increase the severity of CIP. This is partially identical to our study. Previous studies have suggested that subtypes of subpleural fibrosis and honeycomb structures are related to worse prognostic outcomes in lung cancer patients (18,35). Wang et al. (49) found that the presence of GGOs involving multiple lobes on chest CT scans is a risk factor for PFS. In our study, a reticular shadow on chest CT indicated a shorter PFS time (P=0.02) and OS (P=0.02). We did not find that the ILA subtype affected PFS and OS. However, compared to non-SF-ILA patients, SF-ILA patients had shorter PFS and OS, worse prognosis and higher mortality due to CIP-related respiratory failure, according to Kikuchi et al. (48). Due to our limited sample size, only 13 patients had SF-ILA. Of the patients, 11, 8, and 5 had honeycomb structures, traction bronchiectasis, and non-emphysematous cysts, respectively. Thus, due to the limited data, uncertainty remains as to whether the risk of CIP and survival can be predicted using radiological features.
The effect of thoracic radiotherapy on CIP remains unclear. Some studies have reported no association between chest radiotherapy and CIP (38,50), but others have suggested that chest radiotherapy before immunotherapy is a risk factor for CIP (28,29). In our study, chest radiotherapy was not associated with CIP; however, this may be because we mainly studied first-line immunotherapy, and few patients had received chest radiotherapy before ICI therapy.
Inconsistencies exist in the existing literature on the association between PFTs and the occurrence of CIP. For example, Wong et al. reported that reduced total lung capacity (TLC) in patients with solid tumors with pre-existing ILA is associated with CIP (30). However, in another study, no significant correlation was found between CIP and abnormal PFTs at 12 and 18 months before the commencement of ICI therapy in lung cancer patients with ILA (29). In addition, Suzuki et al. investigated the association of FVC, FEV1, TLC, functional residual capacity (FRC), residual volume, and diffusing capacity for carbon monoxide (DLCO) with immune-related ILD (33). Due to objective constraints, we did not have access to DLCO inspection data. In our study, we did not find any PFT parameters that could predict the development of CIP in advanced NSCLC patients receiving first-line immunotherapy. However, this may be because few patients had PFTs before treatment. In the patients with pre-existing ILA, not all the patients had PFT results simultaneously. As a result, we might not have selected the patients most likely to have abnormal PFTs. To gain a better understanding of the role of PFTs in the development of CIP, further prospective research and more PFT data are needed.
The issue of managing the risk of CIP in lung cancer patients with ILA while receiving first-line immunotherapy currently in our study involves three main areas. First, lung cancer patients with ILA who are treated with ICI have a significantly increased risk of CIP, but not all patients with ILA will develop CIP. The severity of ILA needs to be assessed (e.g., extent of fibrosis shown by high-resolution chest CT, lung function indices such as DLCO). If the ILA is mild (e.g., focal GGOs), ICI may still be used with caution; if the risk is high, non-ICI regimens may be considered (e.g., anti-angiogenesis + chemotherapy), especially in those with low PD-L1 expression. For example, the IMpower150 trial (51) showed significant efficacy of bevacizumab + chemotherapy in non-squamous NSCLC, but subgroup data for patients with ILA are lacking. Second, whether patients need prophylactic treatment with low-dose corticosteroids. Steroids can reduce the efficacy of ICIs, and Arbour et al. have shown that hormone combination therapy reduces the efficacy of PD-1 inhibitors (52). Patients with baseline ILA should avoid prophylactic steroids when starting ICIs. Steroid prophylaxis is limited to very high-risk patients and requires individualized decision making. Finally, there is the question of how to determine the frequency of CT monitoring. In patients with asymptomatic ILA, CT is performed every 2–3 ICI cycles (6–9 weeks) in conjunction with lung function (e.g., DLCO, FVC). Low-dose CT after each ICI cycle (every 3–4 weeks) is recommended for early detection of CIP (e.g., GGO or new solid lesions) in those with extensive baseline ILA or a history of acute exacerbation of interstitial pneumonia. Scan immediately at the onset of symptoms (7,53).
Our study had a number of limitations. First, it was a retrospective cohort study. Therefore, the selection bias of confounders in the study might have influenced the results. For example, for lifestyle, genetic and epigenetic, and imaging biases, future studies will need to incorporate multi-omics data (genomic, imaging, clinical phenotypes) and dynamic follow-up, and develop standardized correction frameworks for confounding factors. Second, while there was a reduction in the survival of patients with ILA, the direct effect of ILA on survival was not identified due to an unclear cause of death in some cases. Third, our study was conducted at a single center; thus, the conclusions might not be able to be extended to other centers. Fourth, due to sample size limitations, while ILA was found to be highly significantly correlated with CIP and survival, this result needs to be studied in larger cohorts. Finally, we focused on the occurrence of CIP during first-line immunotherapy. Immunotherapy prior to tumor progression was taken into account. The staging of the tumor in our study was determined by the time the tumor was diagnosed in the hospital, not by the time the immunotherapy was used. We primarily included patients with stage IV and unresectable stage III NSCLC for whom the specific treatment regimen was a combination of molecular testing, patient status, and patient selection. In our article, the follow-up period in our study was not long enough. We did not consider the follow-up of patients who developed CIP, and we plan to validate this next with a detailed study of patients alone and, if necessary, a prospective study of ILA patients. Therefore, future prospective studies with larger sample sizes at multiple centers need to be conducted to verify our conclusions.
Conclusions
ILA was found to be associated with CIP and a shorter survival time in advanced NSCLC patients after PD-1/PD-L1 inhibitor therapy, and severe CIP was also found to be associated with a shorter OS time. Our findings show that closer clinical attention needs to be paid to ILA in the future.
Acknowledgments
None.
Footnote
Reporting Checklist: The authors have completed the REMARK reporting checklist. Available at https://tlcr.amegroups.com/article/view/10.21037/tlcr-2025-150/rc
Data Sharing Statement: Available at https://tlcr.amegroups.com/article/view/10.21037/tlcr-2025-150/dss
Peer Review File: Available at https://tlcr.amegroups.com/article/view/10.21037/tlcr-2025-150/prf
Funding: This work was supported by
Conflicts of Interest: All authors have completed the ICMJE uniform disclosure form (available at https://tlcr.amegroups.com/article/view/10.21037/tlcr-2025-150/coif). The authors have no conflicts of interest to declare.
Ethical Statement: The authors are accountable for all aspects of the work in ensuring that questions related to the accuracy or integrity of any part of the work are appropriately investigated and resolved. The study was conducted in accordance with the Declaration of Helsinki (as revised in 2013) and approved by the Institutional Review Board of Jinling Hospital (No. 2024DZKY-051-01). The requirement of informed consent was waived due to the retrospective nature of this study.
Open Access Statement: This is an Open Access article distributed in accordance with the Creative Commons Attribution-NonCommercial-NoDerivs 4.0 International License (CC BY-NC-ND 4.0), which permits the non-commercial replication and distribution of the article with the strict proviso that no changes or edits are made and the original work is properly cited (including links to both the formal publication through the relevant DOI and the license). See: https://creativecommons.org/licenses/by-nc-nd/4.0/.
References
- Sung H, Ferlay J, Siegel RL, et al. Global Cancer Statistics 2020: GLOBOCAN Estimates of Incidence and Mortality Worldwide for 36 Cancers in 185 Countries. CA Cancer J Clin 2021;71:209-49. [PubMed]
- Robert C. A decade of immune-checkpoint inhibitors in cancer therapy. Nat Commun 2020;11:3801. [PubMed]
- Reck M, Remon J, Hellmann MD. First-Line Immunotherapy for Non-Small-Cell Lung Cancer. J Clin Oncol 2022;40:586-97. [PubMed]
- Ramos-Casals M, Brahmer JR, Callahan MK, et al. Immune-related adverse events of checkpoint inhibitors. Nat Rev Dis Primers 2020;6:38. [PubMed]
- Ramos-Casals M, Sisó-Almirall A. Immune-Related Adverse Events of Immune Checkpoint Inhibitors. Ann Intern Med 2024;177:ITC17-32. [PubMed]
- Wang SJ, Dougan SK, Dougan M. Immune mechanisms of toxicity from checkpoint inhibitors. Trends Cancer 2023;9:543-53. [PubMed]
- Schneider BJ, Naidoo J, Santomasso BD, et al. Management of Immune-Related Adverse Events in Patients Treated With Immune Checkpoint Inhibitor Therapy: ASCO Guideline Update. J Clin Oncol 2021;39:4073-126. [PubMed]
- Hatabu H, Hunninghake GM, Richeldi L, et al. Interstitial lung abnormalities detected incidentally on CT: a Position Paper from the Fleischner Society. Lancet Respir Med 2020;8:726-37. [PubMed]
- Patel AS, Miller E, Regis SM, et al. Interstitial lung abnormalities in a large clinical lung cancer screening cohort: association with mortality and ILD diagnosis. Respir Res 2023;24:49. [Crossref] [PubMed]
- Jin GY, Lynch D, Chawla A, et al. Interstitial lung abnormalities in a CT lung cancer screening population: prevalence and progression rate. Radiology 2013;268:563-71. [Crossref] [PubMed]
- Grant-Orser A, Min B, Elmrayed S, et al. Prevalence, Risk Factors, and Outcomes of Adult Interstitial Lung Abnormalities: A Systematic Review and Meta-Analysis. Am J Respir Crit Care Med 2023;208:695-708. [Crossref] [PubMed]
- Whittaker Brown SA, Padilla M, Mhango G, et al. Interstitial Lung Abnormalities and Lung Cancer Risk in the National Lung Screening Trial. Chest 2019;156:1195-203. [Crossref] [PubMed]
- Cho SW, Jeong WG, Lee JE, et al. Clinical implication of interstitial lung abnormality in elderly patients with early-stage non-small cell lung cancer. Thorac Cancer 2022;13:977-85. [Crossref] [PubMed]
- Hida T, Hata A, Lu J, et al. Interstitial lung abnormalities in patients with stage I non-small cell lung cancer are associated with shorter overall survival: the Boston lung cancer study. Cancer Imaging 2021;21:14. [Crossref] [PubMed]
- Araki T, Dahlberg SE, Hida T, et al. Interstitial lung abnormality in stage IV non-small cell lung cancer: A validation study for the association with poor clinical outcome. Eur J Radiol Open 2019;6:128-31. [Crossref] [PubMed]
- Nishino M, Cardarella S, Dahlberg SE, et al. Interstitial lung abnormalities in treatment-naïve advanced non-small-cell lung cancer patients are associated with shorter survival. Eur J Radiol 2015;84:998-1004. [Crossref] [PubMed]
- Zhu M, Yi J, Su Y, et al. Newly diagnosed non-small cell lung cancer with interstitial lung abnormality: Prevalence, characteristics, and prognosis. Thorac Cancer 2023;14:1874-82. [Crossref] [PubMed]
- Axelsson GT, Putman RK, Aspelund T, et al. The associations of interstitial lung abnormalities with cancer diagnoses and mortality. Eur Respir J 2020;56:1902154. [Crossref] [PubMed]
- Zhang M, Fan Y, Nie L, et al. Clinical Outcomes of Immune Checkpoint Inhibitor Therapy in Patients With Advanced Non-small Cell Lung Cancer and Preexisting Interstitial Lung Diseases: A Systematic Review and Meta-analysis. Chest 2022;161:1675-86. [Crossref] [PubMed]
- Alomaish H, Ung Y, Wang S, et al. Survival analysis in lung cancer patients with interstitial lung disease. PLoS One 2021;16:e0255375. [Crossref] [PubMed]
- Voltolini L, Bongiolatti S, Luzzi L, et al. Impact of interstitial lung disease on short-term and long-term survival of patients undergoing surgery for non-small-cell lung cancer: analysis of risk factors. Eur J Cardiothorac Surg 2013;43:e17-23. [PubMed]
- Seok J, Park S, Yoon EC, et al. Clinical outcomes of interstitial lung abnormalities: a systematic review and meta-analysis. Sci Rep 2024;14:7330. [PubMed]
- Putman RK, Hatabu H, Araki T, et al. Association Between Interstitial Lung Abnormalities and All-Cause Mortality. JAMA 2016;315:672-81. [PubMed]
- Im Y, Chung MP, Lee KS, et al. Impact of interstitial lung abnormalities on postoperative pulmonary complications and survival of lung cancer. Thorax 2023;78:183-90. [Crossref] [PubMed]
- Iwasawa T, Okudela K, Takemura T, et al. Computer-aided Quantification of Pulmonary Fibrosis in Patients with Lung Cancer: Relationship to Disease-free Survival. Radiology 2019;292:489-98. [Crossref] [PubMed]
- Ahn Y, Lee SM, Choi S, et al. Automated CT quantification of interstitial lung abnormality and interstitial lung disease according to the Fleischner Society in patients with resectable lung cancer: prognostic significance. Eur Radiol 2023;33:8251-62. [Crossref] [PubMed]
- Chen X, Li Z, Wang X, et al. Association of pre-existing lung interstitial changes with immune-related pneumonitis in patients with non-small lung cancer receiving immunotherapy. Support Care Cancer 2022;30:6515-24. [Crossref] [PubMed]
- Petranovic M, McDermott S, Mercaldo S, et al. Impact of Baseline Interstitial Lung Abnormalities on Pneumonitis Risk in Patients Receiving Immune Checkpoint Inhibitors for Non-Small-Cell Lung Cancer. Clin Lung Cancer 2023;24:682-688.e5. [Crossref] [PubMed]
- Wong A, Riley M, Zhao S, et al. Association Between Pretreatment Chest Imaging and Immune Checkpoint Inhibitor Pneumonitis Among Patients With Lung Cancer. J Natl Compr Canc Netw 2023;21:1164-1171.e5. [Crossref] [PubMed]
- Wong A, Riley M, Zhao S, et al. Association between pre-treatment chest imaging and pulmonary function abnormalities and immune checkpoint inhibitor pneumonitis. Cancer Immunol Immunother 2023;72:1727-35. [PubMed]
- Hata A, Hino T, Li Y, et al. Traction Bronchiectasis/Bronchiolectasis in Interstitial Lung Abnormality: Follow-up in the COPDGene Study. Am J Respir Crit Care Med 2023;207:1395-8. [PubMed]
- Zhang Y, Wan H, Richeldi L, et al. Reticulation Is a Risk Factor of Progressive Subpleural Nonfibrotic Interstitial Lung Abnormalities. Am J Respir Crit Care Med 2022;206:178-85. [PubMed]
- Suzuki Y, Karayama M, Uto T, et al. Assessment of Immune-Related Interstitial Lung Disease in Patients With NSCLC Treated with Immune Checkpoint Inhibitors: A Multicenter Prospective Study. J Thorac Oncol 2020;15:1317-27. [PubMed]
- Suresh K, Naidoo J, Lin CT, et al. Immune Checkpoint Immunotherapy for Non-Small Cell Lung Cancer: Benefits and Pulmonary Toxicities. Chest 2018;154:1416-23. [Crossref] [PubMed]
- Hata A, Hino T, Yanagawa M, et al. Interstitial Lung Abnormalities at CT: Subtypes, Clinical Significance, and Associations with Lung Cancer. Radiographics 2022;42:1925-39. [Crossref] [PubMed]
- Oldham JM, Adegunsoye A, Khera S, et al. Underreporting of Interstitial Lung Abnormalities on Lung Cancer Screening Computed Tomography. Ann Am Thorac Soc 2018;15:764-6. [Crossref] [PubMed]
- Putman RK, Gudmundsson G, Axelsson GT, et al. Imaging Patterns Are Associated with Interstitial Lung Abnormality Progression and Mortality. Am J Respir Crit Care Med 2019;200:175-83. [Crossref] [PubMed]
- Horiuchi K, Ikemura S, Sato T, et al. Pre-existing Interstitial Lung Abnormalities and Immune Checkpoint Inhibitor-Related Pneumonitis in Solid Tumors: A Retrospective Analysis. Oncologist 2024;29:e108-17. [PubMed]
- Mutsaers SE, Miles T, Prêle CM, et al. Emerging role of immune cells as drivers of pulmonary fibrosis. Pharmacol Ther 2023;252:108562. [PubMed]
- Suresh K, Naidoo J, Zhong Q, et al. The alveolar immune cell landscape is dysregulated in checkpoint inhibitor pneumonitis. J Clin Invest 2019;129:4305-15. [Crossref] [PubMed]
- Mao Z, Pang G, Huang X, et al. Risk factors of immune checkpoint inhibitor-related pneumonitis after neoadjuvant immunochemotherapy for resectable NSCLC. BMC Pulm Med 2024;24:253. [Crossref] [PubMed]
- Daly LE, Power DG, O'Reilly Á, et al. The impact of body composition parameters on ipilimumab toxicity and survival in patients with metastatic melanoma. Br J Cancer 2017;116:310-7. [PubMed]
- Ahmed B, Sultana R, Greene MW. Adipose tissue and insulin resistance in obese. Biomed Pharmacother 2021;137:111315. [Crossref] [PubMed]
- Chu X, Zhao J, Zhou J, et al. Association of baseline peripheral-blood eosinophil count with immune checkpoint inhibitor-related pneumonitis and clinical outcomes in patients with non-small cell lung cancer receiving immune checkpoint inhibitors. Lung Cancer 2020;150:76-82. [Crossref] [PubMed]
- Lin L, Liu Y, Chen C, et al. Association between immune-related adverse events and immunotherapy efficacy in non-small-cell lung cancer: a meta-analysis. Front Pharmacol 2023;14:1190001. [Crossref] [PubMed]
- Li Y, He Y, Chen S, et al. S100A12 as Biomarker of Disease Severity and Prognosis in Patients With Idiopathic Pulmonary Fibrosis. Front Immunol 2022;13:810338. [Crossref] [PubMed]
- Moll M, Peljto AL, Kim JS, et al. A Polygenic Risk Score for Idiopathic Pulmonary Fibrosis and Interstitial Lung Abnormalities. Am J Respir Crit Care Med 2023;208:791-801. [Crossref] [PubMed]
- Kikuchi R, Watanabe Y, Okuma T, et al. Outcome of immune checkpoint inhibitor treatment in non-small cell lung cancer patients with interstitial lung abnormalities: clinical utility of subcategorizing interstitial lung abnormalities. Cancer Immunol Immunother 2024;73:211. [Crossref] [PubMed]
- Wang X, Zhao J, Mei T, et al. Quantification of preexisting lung ground glass opacities on CT for predicting checkpoint inhibitor pneumonitis in advanced non-small cell lung cancer patients. BMC Cancer 2024;24:269. [Crossref] [PubMed]
- Stahlbaum D, Jablonski R, Strek ME, et al. Abnormalities on baseline chest imaging are risk factors for immune checkpoint inhibitor associated pneumonitis. Respir Med 2023;217:107330. [PubMed]
- Socinski MA, Nishio M, Jotte RM, et al. IMpower150 Final Overall Survival Analyses for Atezolizumab Plus Bevacizumab and Chemotherapy in First-Line Metastatic Nonsquamous NSCLC. J Thorac Oncol 2021;16:1909-24. [PubMed]
- Arbour KC, Mezquita L, Long N, et al. Impact of Baseline Steroids on Efficacy of Programmed Cell Death-1 and Programmed Death-Ligand 1 Blockade in Patients With Non-Small-Cell Lung Cancer. J Clin Oncol 2018;36:2872-8. [PubMed]
- Thompson JA, Schneider BJ, Brahmer J, et al. Management of Immunotherapy-Related Toxicities, Version 1.2022, NCCN Clinical Practice Guidelines in Oncology. J Natl Compr Canc Netw 2022;20:387-405. [PubMed]
(English Language Editor: L. Huleatt)