Nomogram based on computed tomography radiomics features and clinicopathological factors to predict the prognosis of patients with non-small cell lung cancer receiving immune checkpoint inhibitor rechallenge
Highlight box
Key findings
• We identified clinicopathological factors and radiomics features that can predict prognosis in patients with advanced non-small cell lung cancer (NSCLC) who received immunotherapy rechallenge following progressive disease (PD) after the first immunotherapy.
What is known and what is new?
• Univariate and multivariate analyses showed that time from the initial immunotherapy to PD occurrence (duration), clinical N stage, liver metastasis, treatment after PD following the first immunotherapy (post-PD treatment), and radiomics features were independent predictive factors for progression-free survival (PFS). In addition, age, duration, clinical N stage, post-PD treatment, and radiomics were independent predictive factors for overall survival (OS).
• The area under the curves of the nomogram for predicting 6-, 12-, and 18-month PFS and 12-, 18-, and 24-month OS were 0.731, 0.809, 0.878, 0.742, 0.782, and 0.868, respectively, in the training cohorts, whereas in the validation cohort were 0.672, 0.774, 0.826, 0.833, 0.705, and 0.762. This indicated good discrimination.
What is the implication, and what should change now?
• We developed and validated a predictive nomogram based on clinicopathological factors and radiomics features for the prognosis of patients with advanced NSCLC who received immunotherapy rechallenge following PD after the first immunotherapy.
Introduction
Background
Immunotherapy has provided a new frontier for lung cancer treatment, with the use of immune checkpoint inhibitors (ICIs) significantly increasing the survival rates among people with progressive lung tumors (1-3). Progressive disease (PD) occurs in the majority of individuals treated with ICI, albeit it may be because immunotherapy is unsuccessful or since resistance develops (4-6). There have been several studies on whether advanced non-small cell lung cancer (NSCLC) that has progressed after the initial immunotherapy can be retreated with immunotherapy. Individuals who experienced a recurrence within 19 months after immunotherapy showed better median overall survival (OS) and 18-month survival rates with reintroduction of immunotherapy than with alternative forms of therapy (6). In the 5-year monitoring of KEYNOTE-024, 12 patients who had progressed after the initial pembrolizumab course demonstrated a 33.3% objective response rate (ORR) and 83.3% disease control rate (DCR) after receiving a subsequent pembrolizumab course (7). With a 42.9% ORR and a 78.6% DCR, 14 sufferers retreated using pembrolizumab following PD in the KEYNOTE-010 trial (8). Additionally, Topp et al. reported that continued pembrolizumab treatment after PD yielded clinical benefits in a subset of patients, with ≥30% of the lesions shrinking in 8.9–24.4% of patients and 64.8–75.9% of patients showing no further lesion progression (9).
However, another study showed that even in patients whose initial ICI treatment was effective, PD occurrence led to limited efficacy of the ICI rechallenge (10).
Rationale and knowledge gap
Therefore, there remains controversy regarding the subset of patients with NSCLC with PD after the initial immunotherapy that can benefit from immunotherapy rechallenge. Accordingly, there is a need for a predictive model based on clinicopathological factors and radiomics features that can help clinicians identify patients who can benefit from immunotherapy after PD. The field of radiomics presents a potential new way to diagnose cancer, forecast lymph node metastases, and assess patient prognosis using quantitative analysis of clinical pictures using highly quantitative characteristics (11,12).
Objective
In this study, we aimed to identify predictive factors among clinicopathological characteristics and radiomics features of primary lung lesions and develop a nomogram to predict the prognosis of patients with NSCLC with PD following immunotherapy who undergo immunotherapy rechallenge. We present this article in accordance with the TRIPOD reporting checklist (available at https://tlcr.amegroups.com/article/view/10.21037/tlcr-24-876/rc).
Methods
Patient selection
Individuals treated with immunotherapy for phase III–IV NSCLC who exhibited PD at the Shandong Cancer Hospital and Institute between 2019 and 2022 were included during this retrospective analysis. Patients had to satisfy three conditions to be included: (I) they had to have a pathologically diagnosed NSCLC, squamous cell carcinoma or adenocarcinoma; (II) they had to be in clinical phase III–IV; and (III) they had experienced PD after initial immunotherapy and then undergone rechallenge with combination immunotherapy. The following were the conditions for rejection: (I) presence of sensitive gene mutations, i.e., epidermal growth factor receptor/anaplastic lymphoma kinase; (II) causing mortality other than tumor; and (III) missing imaging or clinicopathological information and loss to follow-up. Ultimately, this study included 352 patients who were randomly assigned to the training cohort (n=246) or validation cohort (n=106) at a 7:3 ratio (Figure S1). This study was approved by the Ethics Committee of the Shandong Cancer Hospital and Institute (No. SDTHEC202409013) and individual consent for this retrospective analysis was waived. The RECIST v1.1 guidelines were referenced for the definition of PD in this study (13).
Treatment adjuvant
All patients received a rechallenge of immunotherapy combined with chemotherapy (Immuno + Chemo) or antiangiogenic therapy (Immuno + antiangiogenic) after PD following the initial immunotherapy. Administered at intervals of 3 weeks, the immunotherapy regimen included intravenous inhibitors of programmed cell death-1 (PD-1) or programmed cell death-ligand 1 (PD-L1). Chemotherapy regimens included platinum-based drugs, paclitaxel/albumin paclitaxel, and pemetrexed administered through an intravenous route. Among the platinum-based medications, cisplatin was given at a dosage of 25 mg/m2 for 1 to 3 days, whereas carboplatin had an area under the curve (AUC) of 5. The therapies using paclitaxel (135–175 mg/m2) and albumin-bound paclitaxel (260 mg/m2). A dosage of 500 mg/m2 of pemetrexed was administered. Anlotinib (12 mg orally per day for a 2-week accompanied by a week withdrawal phase) and bevacizumab (15 mg/kg intravenously for 3 weeks) were the antiangiogenic treatments.
Study endpoints
The study outcomes were OS and progression-free survival (PFS). PFS was defined as the duration between the post-PD initiation of systemic antitumor therapy and recurrence, death due to cancer, or last follow-up visit. OS was defined as the duration between the post-PD initiation of systemic antitumor therapy and cancer-related death or last follow-up visit.
Radiomics
Computed tomography (CT) scans obtained before initiation of immunotherapy rechallenge were examined. We used 3D Slicer (version 5.2.1) to manually illustrate every tumor target region. Subsequently, another physician with competence in chest CT analysis verified the regions of interest (ROIs). The ROI contained only the primary tumor (Figure S2). To reduce variation between images from different patients, all data were normalized using the Z-score. There was an additional phase for selecting characteristics. In the start, variables were classified by the intraclass correlation coefficient (ICC), retaining only characteristics with an ICC >0.9 to prevent overfitting. Following that, the least absolute shrinkage and selection operator (LASSO) regression approach was used to identify the fundamental characteristics (14,15). Ultimately, a radiomics value (Rad score) was computed for all individuals by attributing values to attributes relying on their coefficients (16).
Prediction and assessment of models
We analyzed clinicopathological factors, including disease stage, post-PD treatment (treatment after PD following the first immunotherapy), duration (time from the start of initial immunotherapy to occurrence of PD), and radiomics information. First, in the training cohort, we studied the prognostic value of radiomics and clinicopathological traits for OS and PFS by univariate analyses. Second, we included factors with P<0.05 in the univariate analyses in the multivariate analyses. Finally, we used significant predictive factors identified in the multivariate Cox regression analyses to construct a nomogram. The nomogram’s predictive ability for PFS and OS in all times was assessed using decision curve analyses (DCAs), calibrating curves, and receiver operating characteristic (ROC) curves.
Statistical analysis
Comparisons of categorical variables were performed using the Chi-squared test or Fisher’s exact test, whereas comparisons of continuous variables were performed using the rank-sum test or independent samples t-test. Both univariate and multivariate studies were done using the Cox proportional hazards models. The Kaplan-Meier method was employed for PFS and OS analysis, followed by a log-rank test. Data processing and visualization were performed using R software 4.3.2, and statistical significance was set at P<0.05.
Results
Patient characteristics
We included 352 patients with stage III–IV NSCLC who received immunotherapy rechallenge. The enrolled participants included 280 (79.5%) males and 72 (20.5%) females; additionally, 173 (49.1%) and 179 (50.9%) patients were aged ≤60 and >60 years, respectively. Among them, 130 (36.9%) patients had squamous cell carcinoma, and 222 (63.1%) had adenocarcinoma. In the training and validation cohorts, death occurred in 196/246 (79.7%) and 84/106 (79.2%) patients, respectively; disease progression occurred in 228 (92.7%) and 99 (93.4%) patients, respectively (Table 1).
Table 1
Characteristics | Training (n=246), n (%) | Validation (n=106), n (%) | χ2 | P |
---|---|---|---|---|
Sex | ||||
Female | 52 (21.1) | 20 (18.9) | 0.23 | 0.63 |
Male | 194 (78.9) | 86 (81.1) | ||
Age | ||||
≤60 years | 118 (48.0) | 55 (51.9) | 0.46 | 0.50 |
>60 years | 128 (52.0) | 51 (48.1) | ||
Smoking | ||||
Yes | 96 (39.0) | 44 (41.5) | 0.19 | 0.66 |
No | 150 (61.0) | 62 (58.5) | ||
Pathology | ||||
Squamous carcinoma | 92 (37.4) | 38 (35.8) | 0.08 | 0.78 |
Adenocarcinoma | 154 (62.6) | 68 (64.2) | ||
Duration | ||||
≤6 months | 129 (52.4) | 55 (51.9) | 0.01 | 0.92 |
>6 months | 117 (47.6) | 51 (48.1) | ||
Immunotherapy drugs | ||||
PD-1 | 227 (92.3) | 96 (90.6) | 0.29 | 0.59 |
PD-L1 | 19 (7.7) | 10 (9.4) | ||
KPS | ||||
≥90 | 104 (42.3) | 43 (40.6) | 0.06 | 0.81 |
<90 | 142 (57.7) | 63 (59.4) | ||
Clinical T stage | ||||
≤2 | 118 (48.0) | 55 (51.9) | 0.46 | 0.50 |
>2 | 128 (52.0) | 51 (48.1) | ||
Clinical N stage | ||||
N+ | 236 (95.9) | 103 (97.2) | 0.07 | 0.80 |
N0 | 10 (4.1) | 3 (2.8) | ||
Clinical TNM stage | ||||
III | 25 (10.2) | 8 (7.5) | 0.60 | 0.44 |
IV | 221 (89.8) | 98 (92.5) | ||
Lymphatic node metastasis* | ||||
Yes | 39 (15.9) | 20 (18.9) | 0.48 | 0.49 |
No | 207 (84.1) | 86 (81.1) | ||
Brain metastasis* | ||||
Yes | 46 (18.7) | 20 (18.9) | 0.00 | 0.97 |
No | 200 (81.3) | 86 (81.1) | ||
Progression of primary lesions* | ||||
Yes | 64 (26.0) | 28 (26.4) | 0.01 | 0.94 |
No | 182 (74.0) | 78 (73.6) | ||
Liver metastasis* | ||||
Yes | 22 (8.9) | 5 (4.7) | 1.87 | 0.17 |
No | 224 (91.1) | 101 (95.3) | ||
Post-PD treatment† | ||||
Immuno + antiangiogenic | 109 (44.3) | 49 (46.2) | 0.11 | 0.74 |
Immuno + Chemo | 137 (55.7) | 57 (53.8) |
*, progression following the first immunotherapy; †, treatment after progression following the first immunotherapy. Duration, time between first immunotherapy and disease progression; PD-1, programmed cell death-1; PD-L1, programmed cell death-ligand 1; KPS, Karnofsky Performance Status; TNM, tumor-node-metastasis; PD, disease progression per RECIST v1.1; Immuno + antiangiogenic, immunotherapy combined with antiangiogenic therapy; Immuno + Chemo, immunotherapy combined with chemotherapy.
Univariate and multivariate analyses
When examining clinicopathological parameters in a univariate way, duration (P<0.001), clinical N stage (P=0.004), liver metastasis (P<0.001), and post-PD treatment (P=0.003) were significantly correlated with PFS (Table 2). Additionally, age (P=0.03), duration (P<0.001), clinical N stage (P=0.008), lymphatic node metastasis (P=0.043), liver metastasis (P=0.01), and post-PD treatment (P<0.001) were significantly associated with OS (Table 3). We selected 12 imaging features that showed the highest predictive utility for PFS. The calculated Rad scores in patients with and without PD were 0.81±1.60 and 2.97±1.51, respectively (P<0.001). Additionally, we selected 15 imaging features that showed the highest predictive utility for OS. The calculated Rad scores in patients who died and survived were 0.40±1.43 and 1.77±1.55, respectively (P<0.001, Figure S3).
Table 2
Characteristics | Univariate analysis | Multivariate analysis | ||||
---|---|---|---|---|---|---|
HR (95% CI) | P value | Regression coefficient | HR (95% CI) | P value | ||
Sex | ||||||
Female | Ref | |||||
Male | 0.84 (0.61–1.16) | 0.29 | ||||
Age | ||||||
≤60 years | 0.91 (0.70–1.18) | 0.47 | ||||
>60 years | Ref | |||||
Smoking | ||||||
Yes | 0.88 (0.68–1.15) | 0.36 | ||||
No | Ref | |||||
Pathology | ||||||
Squamous carcinoma | 0.81 (0.62–1.06) | 0.12 | ||||
Adenocarcinoma | Ref | |||||
Duration | ||||||
≤6 months | 2.05 (1.57–2.68) | <0.001 | 0.60 | 1.83 (1.39–2.40) | <0.001 | |
>6 months | Ref | Ref | Ref | |||
Immunotherapy drugs | ||||||
PD-1 | Ref | |||||
PD-L1 | 0.81 (0.50–1.29) | 0.37 | ||||
KPS | ||||||
≥90 | 0.75 (0.54–1.08) | 0.24 | ||||
<90 | Ref | |||||
Clinical T stage | ||||||
≤2 | 0.96 (0.74–1.24) | 0.75 | ||||
>2 | Ref | |||||
Clinical N stage | ||||||
N+ | Ref | Ref | Ref | |||
N0 | 0.33 (0.15–0.70) | 0.004 | −0.85 | 0.43 (0.20–0.93) | 0.03 | |
Clinical TNM stage | ||||||
III | Ref | |||||
IV | 1.47 (0.95–2.27) | 0.08 | ||||
Lymph node metastasis* | ||||||
Yes | 0.79 (0.55–1.14) | 0.20 | ||||
No | Ref | |||||
Brain metastasis* | ||||||
Yes | 0.99 (0.70–1.39) | 0.95 | ||||
No | Ref | |||||
Progression of primary lesions* | ||||||
Yes | 1.21 (1.02–1.97) | 0.24 | ||||
No | Ref | |||||
Liver metastasis* | ||||||
Yes | 3.32 (2.10–5.25) | <0.001 | 1.03 | 2.81 (1.76–4.48) | <0.001 | |
No | Ref | Ref | Ref | |||
Post-PD treatment† | ||||||
Immuno + antiangiogenic | 0.67 (0.51–0.87) | 0.003 | −0.29 | 0.75 (0.57–0.98) | 0.03 | |
Immuno + Chemo | Ref | Ref | Ref | |||
Rad score | 0.78 (0.71–0.85) | <0.001 | −0.25 | 0.78 (0.72–0.85) | <0.001 |
*, progression following the first immunotherapy; †, treatment after progression following the first immunotherapy. PFS, progression-free survival; HR, hazard ratio; CI, confidence interval; Duration, time interval between the first immunotherapy and disease progression; PD-1, programmed cell death-1; PD-L1, programmed cell death-ligand 1; KPS, Karnofsky Performance Status; TNM, tumor-node-metastasis; PD, disease progression per RECIST v1.1; Immuno + antiangiogenic, immunotherapy combined with antiangiogenic therapy; Immuno + Chemo, immunotherapy combined with chemotherapy; Rad score, radiomics score.
Table 3
Characteristics | Univariate analysis | Multivariate analysis | ||||
---|---|---|---|---|---|---|
HR (95% CI) | P value | Regression coefficient | HR (95% CI) | P value | ||
Sex | ||||||
Female | Ref | |||||
Male | 1.00 (0.70–1.41) | >0.99 | ||||
Age (years) | ||||||
≤60 | 0.71 (0.55–0.97) | 0.03 | −0.16 | 0.79 (0.63–1.13) | 0.03 | |
>60 | Ref | Ref | Ref | |||
Smoking | ||||||
Yes | 0.95 (0.71–1.26) | 0.71 | ||||
No | Ref | |||||
Pathology | ||||||
Squamous carcinoma | 1.13 (0.85–1.51) | 0.40 | ||||
Adenocarcinoma | Ref | |||||
Duration | ||||||
≤6 months | 1.99 (1.48–2.67) | <0.001 | 0.59 | 1.80 (1.33–2.44) | <0.001 | |
>6 months | Ref | Ref | Ref | |||
Immunotherapy drugs | ||||||
PD-1 | Ref | |||||
PD-L1 | 0.63 (0.37–1.09) | 0.10 | ||||
KPS | ||||||
≥90 | 0.82 (0.61–1.12) | 0.45 | ||||
<90 | Ref | |||||
Clinical T stage | ||||||
≤2 | 0.79 (0.60–1.05) | 0.10 | ||||
>2 | Ref | |||||
Clinical N stage | ||||||
N+ | Ref | Ref | Ref | |||
N0 | 0.38 (0.15–0.82) | 0.008 | −0.31 | 0.49 (0.24–0.97) | 0.041 | |
Clinical TNM stage | ||||||
III | Ref | |||||
IV | 1.13 (0.85–1.97) | 0.90 | ||||
Lymph node metastasis* | ||||||
Yes | 0.65 (0.43–0.99) | 0.043 | −0.38 | 0.68 (0.45–1.04) | 0.07 | |
No | Ref | Ref | Ref | |||
Brain metastasis* | ||||||
Yes | 0.94 (0.65–1.37) | 0.75 | ||||
No | Ref | |||||
Progression of primary lesions* | ||||||
Yes | 0.90 (0.65–1.24) | 0.53 | ||||
No | Ref | |||||
Liver metastasis* | ||||||
Yes | 1.80 (1.13–2.87) | 0.01 | 0.12 | 1.12 (0.69–1.82) | 0.64 | |
No | Ref | Ref | Ref | |||
Post-PD treatment† | ||||||
Immuno + antiangiogenic | 0.58 (0.43–0.78) | <0.001 | −0.60 | 0.55 (0.41–0.74) | <0.001 | |
Immuno + Chemo | Ref | Ref | Ref | |||
Rad score | 0.76 (0.69–0.82) | <0.001 | −0.28 | 0.76 (0.69–0.83) | <0.001 |
*, progression following the first immunotherapy; †, treatment after progression following the first immunotherapy. OS, overall survival; HR, hazard ratio; CI, confidence interval; Duration, time interval between the first immunotherapy and disease progression; PD-1, programmed cell death-1; PD-L1, programmed cell death ligand 1; KPS, Karnofsky Performance Status; TNM, tumor-node-metastasis; PD, disease progression per RECIST v1.1; Immuno + antiangiogenic, immunotherapy combined with antiangiogenic therapy; Immuno + Chemo, immunotherapy combined with chemotherapy; Rad score, radiomics score.
Clinicopathological factors and radiomics features with P<0.05 in the univariate analysis were included in the multivariate Cox regression analysis. Multivariate Cox regression analysis revealed the following independent prognostic factors for PFS: duration ≤6 months [hazards ratio (HR): 1.83, 95% confidence interval (CI): 1.39–2.40, P<0.001], clinical N stage N0 (HR: 0.43, 95% CI: 0.20–0.93, P=0.03), liver metastasis (HR: 2.81, 95% CI: 1.76–4.48, P<0.001), post-PD immune + antiangiogenic treatment (HR: 0.75, 95% CI: 0.57–0.98, P=0.03), and Rad score (HR: 0.78, 95% CI: 0.72–0.85, P<0.001). Further, multivariate Cox regression analysis revealed the following independent prognostic factors for OS: age ≤60 years (HR: 0.79, 95% CI: 0.63–1.13, P=0.03), duration ≤6 months (HR: 1.80, 95% CI: 1.33–2.44, P<0.001), clinical N stage N0 (HR: 0.49, 95% CI: 0.24–0.97, P=0.041), post-PD immune + antiangiogenic treatment (HR: 0.55, 95% CI: 0.41–0.74, P<0.001), and Rad score (HR: 0.76, 95% CI: 0.69–0.83, P<0.001).
Establishment and evaluation of the nomogram
We built a predictive nomogram using the significant factors identified in the multivariate Cox regression analyses (Figure 1). To perform the nomogram’s prediction ability, we used ROC curve testing (Figure 2). In the training cohort, the AUC values of the nomogram for predicting 6-, 12-, and 18-month PFS were 0.731 (95% CI: 0.664–0.798), 0.809 (95% CI: 0.748–0.870), and 0.878 (95% CI: 0.800–0.956), respectively, whereas those for predicting 12-, 18-, and 24-month OS were 0.742 (95% CI: 0.673–0.811), 0.782 (95% CI: 0.723–0.842), and 0.868 (95% CI: 0.779–0.956), respectively. All these AUC values were higher than those for single predictive factors (Tables S1,S2). In the validation cohort, the AUC values of the nomogram for predicting 6-, 12-, and 18-month PFS were 0.672 (95% CI: 0.574–0.784), 0.774 (95% CI: 0.677–0.864), and 0.826 (95% CI: 0.734–0.962), respectively, whereas those for predicting 12-, 18-, and 24-month OS were 0.833 (95% CI: 0.738–0.929), 0.705 (95% CI: 0.599–0.811), and 0.762 (95% CI: 0.641–0.882), respectively (Tables S1,S2). Furthermore, there was good agreement among the expected and actual PFS and OS in the calibrating curves of the validation and training sets of both prediction models (Figures S4,S5). For both forecasting models, DCA exhibited a significant positive effect across the majority of criterion probability, indicating their high clinical applicability (Figures S6,S7).
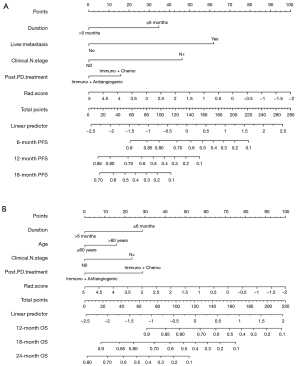
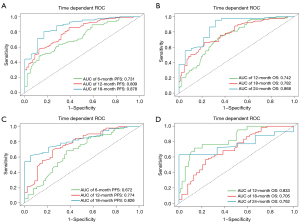
Assessing model for predictive stratification
The X-tile algorithm has been applied to calculate cutoff metrics for all participants based on the overall score obtained from the nomogram to assess PFS and OS. A minimal-risk group (n=302, overall score ≤169.7; n=153, total score ≤127.8) and a higher-risk group (n=50, total score >169.7; n=199, entire score >127.8) have been identified based on such parameters. The two populations differed significantly, indicating considerable stratification, based on KM survival analyses. The minimal-risk group’s 6-, 12-, and 18-month PFS rates were considerably greater than those of the high-risk group (66.2% vs. 24.0%, 27.9% vs. 0.0%, and 4.3% vs. 0.0%, respectively; P<0.001). The OS rates at 12, 18, and 24 months also showed significant differences (86.1% vs. 57.1%, 61.6% vs. 23.8%, and 23.3% vs. 5.2%, respectively; P<0.001) (Figure 3).
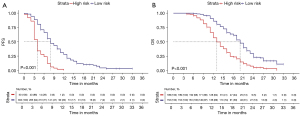
Discussion
Key findings
Immunotherapy has become widely used and has facilitated the treatment of patients with advanced NSCLC. However, a large proportion of patients inevitably develop immunoreactivity (6). Our previous studies have shown that, patients with NSCLC experiencing tumor progression post-immunotherapy can still benefit from further treatment, with immunotherapy combined with antiangiogenic therapy being the most efficacious option (17). However, it remains unclear which patients will benefit from immunotherapy rechallenge. Therefore, identifying the subset of these patients with PD who can still benefit from subsequent immunotherapy is a pressing clinical issue. Therefore, using clinicopathological variables and CT radiomics attributes, we created and tested an initial diagnostic model for OS and PFS. Our developed nomogram could effectively predict patients with advanced NSCLC who can still benefit from immunotherapy rechallenge, which could inform clinical treatment strategies.
Using multivariate analysis, we found that duration, clinical N stage, liver metastasis, post-PD treatment, and Rad score were independent predictive factors for PFS among patients with NSCLC who received immunotherapy rechallenge; moreover, age, duration, clinical N stage, post-PD treatment, and Rad score were independent predictive factors for OS among these patients. The resulting model had high AUC values in both the training and validation cohorts, which were higher than those for each single predictive factor, demonstrating the high predictive utility of our nomogram.
Furthermore, this study analyzed the impact of different progressions after the first immunotherapy on the efficacy of subsequent immunotherapy and found that the development of liver metastases after the first immunotherapy was an independent risk factor for PFS. Therefore, immunotherapy rechallenge is not recommended for patients with clinical N stage N+ and liver metastases after the first immunotherapy.
Strengths and limitations
There are some limitations in this study. First, the retrospective design of this study may have led to selection bias. Second, not all of the disease progression that occurred after first-line immunotherapy in this study was confirmed pathologically. Third, the PD-L1 expression level of each patient could not be collected in this study; therefore, we did not include the PD-L1 expression level of the patients in the analysis.
Comparison with similar researches
Our findings revealed a significant prognostic advantage for patients with a >6-month duration interval from the start of the first immunotherapy to PD occurrence, which is possibly linked to the effectiveness of the first therapy. This confirms prior studies indicating that even if a patient develops PD, they may still benefit from a follow-up immunotherapy challenge (6,7,18).
In our study, patients were classified into the Immuno + Chemo and Immuno + antiangiogenic groups based on the immunotherapy combination regimen, with the Immuno + antiangiogenic group showing a significant survival advantage. Previous studies have suggested that the combined application of immunotherapy and antiangiogenic therapy has a strong synergistic effect (19-21).
In addition, our results showed that patients with N+ clinical N stage had poorer PFS and OS following immunotherapy rechallenge. Our results are supported by earlier research showing that tumor cells that spread to lymphatic nodes inhibit the proliferation of immune cells in the nodes, hence decreasing the efficacy of immunotherapy (22). A study by Deng et al. hypothesized that the quantity of memory T lymphocytes in lymphatic nodes affects the success of immunotherapy (23).
Several studies have explored the predictive utility of CT-based radiomics for treatment response and prognosis (12,24,25). Liu et al. retrospectively analyzed 89 patients with NSCLC who received neoadjuvant chemotherapy in combination with immunotherapy, and they found that preprocessed CT images and clinical features could be used to effectively predict major pathological remission (26). Moreover, Khorrami et al. used textural features within and outside tumor nodes to effectively predict the treatment response and OS of patients with NSCLC treated with ICI therapy (27). Using radiomics to find individuals with advanced NSCLC who may still benefit from immunotherapy rechallenge following PD after the initial immunotherapy is a plausible hypothesis.
Explanations of findings
The Society for Immunotherapy of Cancer distinguishes among three scenarios of immunotherapy resistance: primary resistance, secondary resistance, and progression after therapy discontinuation for any reason (28,29). The tumor microenvironment (30), signaling pathways (31), and gene deletions (32) are associated with primary drug resistance. The mechanisms driving secondary resistance may involve upregulation of other immune checkpoints, genetic defects, or genetic mutations (33,34).
A complex relationship exists between tumor angiogenesis and immunity. A number of pro-angiogenic substances are discharged by tumor and stromal tissues in response to hypoxia in the tumor microenvironment, which causes aberrant arteries development (35). The formation of this pathological vasculature within the tumor, which is tortuous and highly permeable, can lead to a hypoxic and acidic tumor microenvironment. This impedes the infiltration of immune effector cells and ultimately creates an immunosuppressive microenvironment (36,37). Anti-angiogenic drugs inhibit the formation of this pathological vasculature, leading to increased T-cell infiltration, and thus enhances the immune response (35). Low apatinib doses in a mouse model of lung cancer increase T-cell infiltration and decrease the tumor recruitment of relevant suppressor immune cells (38). Vascular endothelial growth factor (VEGF) binds to VEGF receptor-2 on dendritic cells (DCs), which increases PD-1 expression, inhibits DC development, and causes a substantial decrease in their antigen-presenting capacity (39). Anti-angiogenic drugs attenuate the inhibitory effect of VEGF on DCs, and thus enhance the presentation of tumor antigens by DCs. Chu et al. reported that sintilimab + anlotinib combination therapy achieved an encouraging ORR of 72.7% and DCR of 100% (40). Similarly, pembrolizumab + ramucirumab combination therapy has superior efficacy in treating advanced solid tumors (41). Taken together, patients with NSCLC who present PD following immunotherapy may experience increased clinical benefit from rechallenge with immunotherapy combined with antiangiogenic therapy.
Additionally, the risk score for each patient was calculated using a nomogram, and different risk thresholds in the nomogram were obtained using X-Tile software. Survival analysis among the different risk subgroups revealed that the scores, which represent the risk, were negatively correlated with the prognosis of patients who experience PD after the first immunotherapy.
Implications and actions needed
Further research is warranted to clarify the mechanisms underlying the development of immunotherapy resistance and explore predictive markers for identifying patients who may experience therapeutic benefits. For patients considered by our prediction model to be at high risk, we do not recommend immunotherapy rechallenge after PD. Moreover, although low-risk patients may still benefit from immunotherapy, they should undergo regular review with close scrutiny for changes in various risk factors.
Conclusions
In this study, we developed and validated a nomogram based on clinicopathological factors and radiomics features that showed strong predictive utility for the prognosis of patients with advanced NSCLC who are rechallenged with immunotherapy after PD occurrence following the first immunotherapy.
Acknowledgments
None.
Footnote
Reporting Checklist: The authors have completed the TRIPOD reporting checklist. Available at https://tlcr.amegroups.com/article/view/10.21037/tlcr-24-876/rc
Data Sharing Statement: Available at https://tlcr.amegroups.com/article/view/10.21037/tlcr-24-876/dss
Peer Review File: Available at https://tlcr.amegroups.com/article/view/10.21037/tlcr-24-876/prf
Funding: This work was supported by
Conflicts of Interest: All authors have completed the ICMJE uniform disclosure form (available at https://tlcr.amegroups.com/article/view/10.21037/tlcr-24-876/coif). The authors have no conflicts of interest to declare.
Ethical Statement: The authors are accountable for all aspects of the work in ensuring that questions related to the accuracy or integrity of any part of the work are appropriately investigated and resolved. The study was conducted in accordance with the Declaration of Helsinki (as revised in 2013). This study was approved by the Ethics Committee of the Shandong Cancer Hospital and Institute (No. SDTHEC202409013) and individual consent for this retrospective analysis was waived.
Open Access Statement: This is an Open Access article distributed in accordance with the Creative Commons Attribution-NonCommercial-NoDerivs 4.0 International License (CC BY-NC-ND 4.0), which permits the non-commercial replication and distribution of the article with the strict proviso that no changes or edits are made and the original work is properly cited (including links to both the formal publication through the relevant DOI and the license). See: https://creativecommons.org/licenses/by-nc-nd/4.0/.
References
- Reck M, Remon J, Hellmann MD. First-Line Immunotherapy for Non-Small-Cell Lung Cancer. J Clin Oncol 2022;40:586-97.
- Lahiri A, Maji A, Potdar PD, et al. Lung cancer immunotherapy: progress, pitfalls, and promises. Mol Cancer 2023;22:40.
- Mino-Kenudson M, Schalper K, Cooper W, et al. Predictive Biomarkers for Immunotherapy in Lung Cancer: Perspective From the International Association for the Study of Lung Cancer Pathology Committee. J Thorac Oncol 2022;17:1335-54.
- Xu M, Hao Y, Shi Z, et al. Efficacy of rechallenge immunotherapy after immune monotherapy resistance in patients with advanced non-small cell lung cancer. J Cancer Res Clin Oncol 2023;149:17987-95.
- Xu S, Shukuya T, Tamura J, et al. Heterogeneous Outcomes of Immune Checkpoint Inhibitor Rechallenge in Patients With NSCLC: A Systematic Review and Meta-Analysis. JTO Clin Res Rep 2022;3:100309.
- Gandara DR, von Pawel J, Mazieres J, et al. Atezolizumab Treatment Beyond Progression in Advanced NSCLC: Results From the Randomized, Phase III OAK Study. J Thorac Oncol 2018;13:1906-18.
- Reck M, Rodríguez-Abreu D, Robinson AG, et al. Five-Year Outcomes With Pembrolizumab Versus Chemotherapy for Metastatic Non-Small-Cell Lung Cancer With PD-L1 Tumor Proportion Score ≥ 50. J Clin Oncol 2021;39:2339-49.
- Herbst RS, Garon EB, Kim DW, et al. Long-Term Outcomes and Retreatment Among Patients With Previously Treated, Programmed Death-Ligand 1‒Positive, Advanced Non‒Small-Cell Lung Cancer in the KEYNOTE-010 Study. J Clin Oncol 2020;38:1580-90.
- Topp BG, Channavazzala M, Mayawala K, et al. Tumor dynamics in patients with solid tumors treated with pembrolizumab beyond disease progression. Cancer Cell 2023;41:1680-1688.e2.
- Akamatsu H, Teraoka S, Takamori S, et al. Nivolumab Retreatment in Non-Small Cell Lung Cancer Patients Who Responded to Prior Immune Checkpoint Inhibitors and Had ICI-Free Intervals (WJOG9616L). Clin Cancer Res 2022; Epub ahead of print. [Crossref]
- Braman N, Prasanna P, Whitney J, et al. Association of Peritumoral Radiomics With Tumor Biology and Pathologic Response to Preoperative Targeted Therapy for HER2 (ERBB2)-Positive Breast Cancer. JAMA Netw Open 2019;2:e192561.
- Wang X, Zhao X, Li Q, et al. Can peritumoral radiomics increase the efficiency of the prediction for lymph node metastasis in clinical stage T1 lung adenocarcinoma on CT? Eur Radiol 2019;29:6049-58.
- Schwartz LH, Seymour L, Litière S, et al. RECIST 1.1 - Standardisation and disease-specific adaptations: Perspectives from the RECIST Working Group. Eur J Cancer 2016;62:138-45.
- Zhao W, Wu Y, Xu Y, et al. The Potential of Radiomics Nomogram in Non-invasively Prediction of Epidermal Growth Factor Receptor Mutation Status and Subtypes in Lung Adenocarcinoma. Front Oncol 2019;9:1485.
- Zhao W, Xu Y, Yang Z, et al. Development and validation of a radiomics nomogram for identifying invasiveness of pulmonary adenocarcinomas appearing as subcentimeter ground-glass opacity nodules. Eur J Radiol 2019;112:161-8.
- Liu Z, Zhang XY, Shi YJ, et al. Radiomics Analysis for Evaluation of Pathological Complete Response to Neoadjuvant Chemoradiotherapy in Locally Advanced Rectal Cancer. Clin Cancer Res 2017;23:7253-62.
- Li Y, Zhao J, Li R, et al. Treatment options for tumor progression after initial immunotherapy in advanced non-small cell lung cancer: A real-world study. Neoplasia 2024;57:101043.
- Waterhouse DM, Garon EB, Chandler J, et al. Continuous Versus 1-Year Fixed-Duration Nivolumab in Previously Treated Advanced Non-Small-Cell Lung Cancer: CheckMate 153. J Clin Oncol 2020;38:3863-73.
- Lee CH, Shah AY, Rasco D, et al. Lenvatinib plus pembrolizumab in patients with either treatment-naive or previously treated metastatic renal cell carcinoma (Study 111/KEYNOTE-146): a phase 1b/2 study. Lancet Oncol 2021;22:946-58.
- Provencio M, Ortega AL, Coves-Sarto J, et al. Atezolizumab Plus Bevacizumab as First-line Treatment for Patients With Metastatic Nonsquamous Non-Small Cell Lung Cancer With High Tumor Mutation Burden: A Nonrandomized Controlled Trial. JAMA Oncol 2023;9:344-53.
- Manegold C, Dingemans AC, Gray JE, et al. The Potential of Combined Immunotherapy and Antiangiogenesis for the Synergistic Treatment of Advanced NSCLC. J Thorac Oncol 2017;12:194-207.
- He M, He Q, Cai X, et al. Intratumoral tertiary lymphoid structure (TLS) maturation is influenced by draining lymph nodes of lung cancer. J Immunother Cancer 2023;11:e005539.
- Deng H, Zhou J, Chen H, et al. Impact of lymphadenectomy extent on immunotherapy efficacy in postresectional recurred non-small cell lung cancer: a multi-institutional retrospective cohort study. Int J Surg 2024;110:238-52.
- Nam JG, Park S, Park CM, et al. Histopathologic Basis for a Chest CT Deep Learning Survival Prediction Model in Patients with Lung Adenocarcinoma. Radiology 2022;305:441-51.
- Perez-Johnston R, Araujo-Filho JA, Connolly JG, et al. CT-based Radiogenomic Analysis of Clinical Stage I Lung Adenocarcinoma with Histopathologic Features and Oncologic Outcomes. Radiology 2022;303:664-72.
- Liu C, Zhao W, Xie J, et al. Development and validation of a radiomics-based nomogram for predicting a major pathological response to neoadjuvant immunochemotherapy for patients with potentially resectable non-small cell lung cancer. Front Immunol 2023;14:1115291.
- Khorrami M, Prasanna P, Gupta A, et al. Changes in CT Radiomic Features Associated with Lymphocyte Distribution Predict Overall Survival and Response to Immunotherapy in Non-Small Cell Lung Cancer. Cancer Immunol Res 2020;8:108-19.
- Kluger HM, Tawbi HA, Ascierto ML, et al. Defining tumor resistance to PD-1 pathway blockade: recommendations from the first meeting of the SITC Immunotherapy Resistance Taskforce. J Immunother Cancer 2020;8:e000398.
- Sharma P, Hu-Lieskovan S, Wargo JA, et al. Primary, Adaptive, and Acquired Resistance to Cancer Immunotherapy. Cell 2017;168:707-23.
- Nagarsheth N, Peng D, Kryczek I, et al. PRC2 Epigenetically Silences Th1-Type Chemokines to Suppress Effector T-Cell Trafficking in Colon Cancer. Cancer Res 2016;76:275-82.
- Chen N, Fang W, Zhan J, et al. Upregulation of PD-L1 by EGFR Activation Mediates the Immune Escape in EGFR-Driven NSCLC: Implication for Optional Immune Targeted Therapy for NSCLC Patients with EGFR Mutation. J Thorac Oncol 2015;10:910-23.
- Gao J, Shi LZ, Zhao H, et al. Loss of IFN-γ Pathway Genes in Tumor Cells as a Mechanism of Resistance to Anti-CTLA-4 Therapy. Cell 2016;167:397-404.e9.
- O'Donnell JS, Teng MWL, Smyth MJ. Cancer immunoediting and resistance to T cell-based immunotherapy. Nat Rev Clin Oncol 2019;16:151-67.
- Zaretsky JM, Garcia-Diaz A, Shin DS, et al. Mutations Associated with Acquired Resistance to PD-1 Blockade in Melanoma. N Engl J Med 2016;375:819-29.
- Ramjiawan RR, Griffioen AW, Duda DG. Anti-angiogenesis for cancer revisited: Is there a role for combinations with immunotherapy? Angiogenesis 2017;20:185-204.
- Maeda H, Wu J, Sawa T, et al. Tumor vascular permeability and the EPR effect in macromolecular therapeutics: a review. J Control Release 2000;65:271-84.
- Huang Y, Goel S, Duda DG, et al. Vascular normalization as an emerging strategy to enhance cancer immunotherapy. Cancer Res 2013;73:2943-8.
- Zhao S, Ren S, Jiang T, et al. Low-Dose Apatinib Optimizes Tumor Microenvironment and Potentiates Antitumor Effect of PD-1/PD-L1 Blockade in Lung Cancer. Cancer Immunol Res 2019;7:630-43.
- Zhao Y, Guo S, Deng J, et al. VEGF/VEGFR-Targeted Therapy and Immunotherapy in Non-small Cell Lung Cancer: Targeting the Tumor Microenvironment. Int J Biol Sci 2022;18:3845-58.
- Chu T, Zhong R, Zhong H, et al. Phase 1b Study of Sintilimab Plus Anlotinib as First-line Therapy in Patients With Advanced NSCLC. J Thorac Oncol 2021;16:643-52.
- Herbst RS, Arkenau HT, Bendell J, et al. Phase 1 Expansion Cohort of Ramucirumab Plus Pembrolizumab in Advanced Treatment-Naive NSCLC. J Thorac Oncol 2021;16:289-98.