Persistent and novel changes in plasma microRNA profiles in patients with non-small cell lung cancer following tumour resection
Highlight box
Key findings
• The pre-surgery plasma microRNA profile in untreated non-small cell lung cancer (NSCLC) patients significantly differs from that of normal blood donors, with distinct pattern in adenocarcinoma versus squamous cell carcinoma patients. Some of the pre-surgery alterations may have a potential of a marker of recurrence. After tumour excision plasma microRNA profiles change, some microRNA levels normalise, but the majority of pre-surgery changes persist, suggesting systemic abnormalities predisposing to lung cancer and/or systemic response to pre-cancer/dormant cancer cells. Interestingly, novel microRNA changes are observed following long-term follow-up in patients with no clinical symptoms of recurrence.
What is known and what is new?
• The alterations in plasma microRNA levels in NSCLC patients have been reported previously, however only limited data are available on circulating microRNAs long-term after tumor excision, especially in patients with no clinical symptoms of recurrence.
• To our knowledge this is the first analysis of post-surgery plasma microRNA profiles in NSCLC patients who showed no clinical recurrence following long-term follow-up.
What is the implication, and what should change now?
• Our observations support the rationale for the development of new therapeutic strategies targeting both cancer cell-autonomous and non-autonomous effectors in the tumour ecosystem.
Introduction
Lung cancer is the leading cause of cancer morbidity and mortality, accounting for 12.4% of all cancer diagnoses and responsible for 18.7% of cancer-related deaths. Lung cancer ranks first in incidence and mortality among men and second (after breast cancer) in women. The overall 5-year survival rate of lung cancer patients remains low (<20%), primarily because most cases are diagnosed at an advanced stage. The incidence of lung cancer varies by gender (male: 15.3%, female: 9.4%) (1), and by age, with 47% of lung cancer patients being ≥70 years old and 15% being ≥80 years old (2). Non-small cell lung cancer (NSCLC) accounts for approximately 85% of all lung cancer cases. Factors such as tobacco and environmental exposures (especially asbestos and air pollution), also significantly increase lung cancer risk (1,3).
Tumour resection is the primary treatment for early-stage NSCLC. However, approximately 40% of patients experience recurrence after surgery, mainly due to distant metastases (4-6). Therefore, a better understanding of the molecular mechanisms underlying NSCLC progression is urgently needed to develop new diagnostic approaches for early NSCLC diagnosis and monitoring. Various blood-based approaches have been developed to improve lung cancer diagnosis, including evaluation of circulating cell-free DNA and RNA [including microRNAs (miRs), long non-coding RNAs (lncRNA), messenger RNAs (mRNAs)], circulating extracellular vesicles, tumour-educated platelets, proteins, and metabolites (7,8). Circulating miRs have become a significant focus in lung cancer research in recent years. They are linked to cancer development, progression, and response to treatment. They have the potential to serve as diagnostic and prognostic biomarkers. Additionally, they are involved in the regulation of inflammatory processes and key epigenetic mechanisms (9).
Primary malignant tumours influence not only their nearby stroma, the microenvironment, but also the macroenvironment, by inducing various alterations in benign parts of the organ of origin, in the regional lymph nodes, and in the distant tissues or organs. Some of the systemic cancer-driven changes are associated with tumour aggressiveness and outcome (10-13). Increasing data also show that the host macroenvironment, including intestinal microbiota, metabolism, tensional homeostasis, morphostasis, tissue bioelectric properties, and nervous system, play a crucial role in cancer progression (14).
Changes in circulating miRs following NSCLC resection have been previously analysed, but in all studies, except one (15), post-surgical levels of circulating miRs were analysed shortly after tumour resection (1–2 weeks) (15-17), and may be significantly related to surgical treatment and wound healing. Long-term systemic miR changes seem biologically and clinically important. Our previously published data suggested that deregulated plasma miR-9-5p, miR-16-5p, miR-205-5p, and miR-486-5p levels may reflect not only the presence of primary NSCLC, but also a systemic response to the disease (18). Our current study extends these findings by analysing global plasma miRs in NSCLC patients before and at least one year after tumour resection with a focus on patients showing no clinical evidence of recurrence during long-term follow-up. We present the following article in accordance with the MDAR reporting checklist (available at https://tlcr.amegroups.com/article/view/10.21037/tlcr-24-626/rc).
Methods
Patients and samples
Peripheral blood samples were collected at the Maria Sklodowska-Curie National Research Institute of Oncology in Warsaw from previously untreated patients with histologically confirmed NSCLC, undergoing surgery at our institution between 2011 and 2015. Patients with concomitant lung diseases and those with other tumours were excluded. Finally, out of initial over 100 patients only 36 met our inclusion criteria [group BSAll, median age 62.5 (range, 43–77) years, 23 men and 13 women] (Figure 1, Table S1). Blood samples from donors without cancer [group C, n=24, median age 58.5 (range, 36–70) years, 15 men and 9 women] were also taken (Table S2). The histopathological diagnosis was confirmed as adenocarcinoma (ADC) (n=18) and squamous cell carcinoma (SCC) (n=18), according to the 2021 World Health Organization (WHO) classification (19). Blood was collected from all NSCLC patients one day before surgery (BSAll, n=36, including BSPaired, n=12) and in 12 patients also at least 1 year (2 years on average) after surgery (ASPaired, n=12, ADC n=7 and SCC n=5, median age 61 (range, 43–76) years, 8 men and 4 women) (Figure 1). The patients from the ASPaired group had not received any additional treatment before the second blood sample collection. In 24 patients, blood samples were either not analysed or not collected after surgery (AS). These included samples from patients with serious concomitant diseases or recurrences, and individuals who did not consent to the second collection or continued their follow-up at a different facility. Some samples were also excluded due to pre-analytical reasons. To assess disease recurrence, patients were followed-up for 4 to 12 years after surgery (Table S1). The study was conducted in accordance with the Declaration of Helsinki (as revised in 2013). The study was approved by Bioethics Committee of Maria Sklodowska-Curie National Research Institute of Oncology in Warsaw (No. 4/2011/1/2012), and written informed consent was obtained from sample donors.
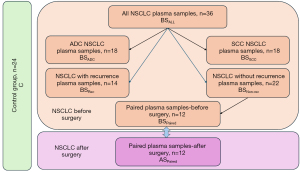
RNA extraction
Total RNA was isolated from plasma samples (300 µL) using mirVana PARIS RNA and Native Protein Purification Kit (Thermo Fisher Scientific, Waltham, MA USA), according to the protocols described earlier by Sromek et al. (18), and stored at −70 ℃.
Next-generation sequencing (NGS)
Small RNA fractions were checked for concentration and quality on Bioanalyzer 2100 using a Small RNA Kit Chip (Agilent, Santa Clara, CA, USA), and assessed with Qubit RNA HS Assay Kits (Thermo Fisher Scientific). miR libraries were prepared from 1 µg of total RNA with an Ion Total RNA-Seq Kit v2 for Small RNA Libraries and Ion Xpress RNA-Seq Barcode Kit (Thermo Fisher Scientific), according to the manufacturer’s protocol. Briefly, reverse transcription of RNA, subsequent complementary DNA (cDNA) purification, and library size selection were performed with Nucleic Acid Binding Beads. Next, cDNA was amplified by polymerase chain reaction (PCR), purified and size selected. For sample quality control, the yield and size distribution DNA constructs were then assessed on the Agilent 2100 Bioanalyzer system using a High Sensitivity DNA Kit (Agilent, Santa Clara, CA, USA). The length of ligation products in barcoded libraries of miR ranged between 94 and 114 bp.
Template preparation procedure for clonal amplification of up to 16 miR libraries at a concentration of 18 pM and loading of the Ion PI Chip v3 were achieved using the Ion Chef instrument, with Ion PITM Hi-QTM Chef Kit (Thermo Fisher Scientific). Sequencing was performed using the Ion Torrent Proton sequencer (Thermo Fisher Scientific).
Reverse transcription-quantitative polymerase chain reaction (RT-qPCR)
The reverse transcription reaction was performed for selected 8 up-regulated and 7 down-regulated miRs with the TaqMan Advanced miR cDNA Synthesis Kit (Applied Biosystems CN A28007) (Table S3). qPCR was performed using the TaqMan Fast Advanced Master Mix (Applied Biosystems Carlsbad, CA USA) on QuantStudioTM 7 Flex Real Time PCR System (Thermo Fisher Scientific). Specific TaqMan Advanced miR Assays (Applied Biosystems Carlsbad, CA USA) were used to measure miRs specified in Table S3. miR-101 and miR-361 were selected as internal controls based on the NormFinder algorithm. All qPCR reactions were carried out in triplicates, and the relative miR quantities were calculated with the 2−∆Ct method. The data were analysed with the QuantStudioTM Real-Time PCR Software (Thermo Fisher Scientific). The differences in miR levels between samples revealed by the RT-qPCR were assessed by the Mann-Whitney U test.
Statistical analysis
The miRDeep2 application was used to map NGS reads to the human genome hg19, quantify the known miRs (miRBase V21/22), and predict novel miRs. Differential miR expression was analysed using the DESeq2 package, with a significance threshold set to 0.05 without the Benjamini-Hochberg correction. The obtained gene expression data were normalised (according to the internal DESeq2 normalisation mechanisms) and, after log2-transformation, used for principal component analysis (PCA) and hierarchical clustering in the R environment, to identify similarities in miR expression patterns between the samples.
The lists of genes with significantly altered expression levels between the studied groups were subsequently used in ontological analyses performed with the miR enrichment analysis and annotation tool (miEAA, https://ccb-compute2.cs.uni-saarland.de/mieaa/, version 2.1). This tool was employed to perform the over-representation analysis of mature miRs in 28 different categories (Table S4). The enrichment analysis was performed with the nonparametric Kolmogorov-Smirnov (KS) test. The minimum hits per sub-category threshold was set to 2. The term was classified as enriched if the KS test P value after the Benjamini-Hochberg adjustment was lower than 0.05. The enriched ontological terms were visualised in word clouds, and the statistical significance of each term along with the over-represented miRs were shown on heatmaps.
A list of 125 miRs identified in the present study as differentially expressed was subjected to detailed statistical inference in the R environment (v. 4.0.2). We used univariable and multivariable Cox proportional hazard models (package: survival, v. 3.5.7) to assess the value of these miRs as potential novel prognostic biomarkers. In each model, overall survival/death and disease-free survival/relapse were used as dependent variables. All Cox models were also checked with respect to the proportionality of hazards for each variable used. The same list of miRs was utilised to assess the risk of altered expression on the development of NSCLC for the tumours compared to the control group, by generating univariable and multivariable logistic regression models (packages: stats., v. 4.0.2, and rms, v. 6.0.1). In these tests, the disease status (presence vs. absence) was utilised as the dependent variable. In all regression models, the normalised miR expression, histological type, grade, and clinical stage of the tumours along with the patient gender and age were used as independent variables. In order to verify the discriminating capabilities of the created regression models, we performed their cross-validation in new datasets, generated from the original data by bootstrapping (with replacement) and a subsequent comparison of the areas under receiver operating characteristic (ROC) curve (AUCs) between the original and bootstrapped datasets, using the riskRegression package (v. 2023.12.21) (20). Each miR was considered a promising predictor if its P value was <0.05 and all the AUC values of the corresponding uni- and multivariable models was >2/3.
Results
Pre-surgery plasma miR levels in NSCLC patients
The entire analysed group of NSCLC patients (BSAll; n=36) comprised those with and without recurrence (BSRec; n=14 and BSNon-rec; n=22, respectively). Sixty-five miRs were significantly differentially expressed between group BSAll and blood donors without cancer (C; n=24), including 48 miRs with higher and 17 miRs with lower expression levels in BSAll than in C group (Figure 2, Table S5). To assess the diagnostic significance of changes in plasma miR levels in NSCLC patients, logistic regression analysis was performed on 65 previously selected plasma miRs that distinguish NSCLC patients from the control group. The analysis using the logistic regression model, which included gender, smoking status, and age, showed the significance of 3 up-regulated (miR-185-5p, miR-423-5p, miR-590-5p) and 5 down-regulated (miR-122b-3p, miR144-3p, miR-20a-5p, miR-20b-5p, miR-451a) miRs as markers associated with cancer (Figure 3, Tables S5,S6). Some miRs from this group also proved to be strong prognostic factors for disease-free survival (DFS) and overall survival (OS), based on Cox regression analysis. Increased levels of miR-21-5p and miR-92a-3p influenced DFS, while decreased levels of miR-122b-3p and miR-150-5p and increased level of miR 92a-3p were linked to an elevated risk of death in patients with NSCLC (Table S7).
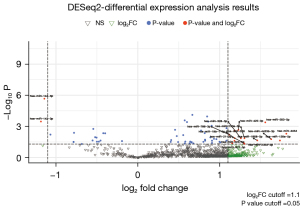
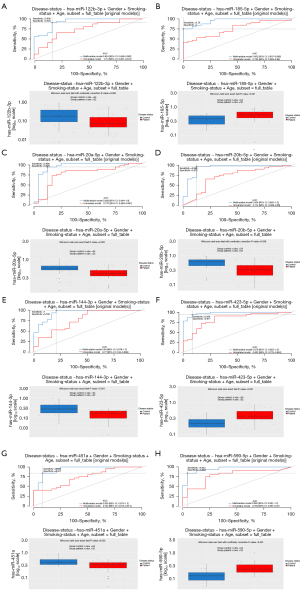
The analysis of pre-surgery plasma miR profiles according to the NSCLC histopathological subtypes (BSADC; n=18 and BSSCC; n=18) showed that the expression of some miRs was significantly altered regardless of the histopathological subtype, while other miR changes depended on the specific subtype. Among those related to histopathological subtypes, there were 9 up-regulated and 10 down-regulated miRs identified as ADC-specific (Figure 4, Figure S1, Table S8), and 79 up-regulated and 11 down-regulated miRs identified as SCC-specific (Figure 4, Figure S2, Table S9). The direct comparison (BSADC vs. BSSCC) of the two histopathological subtypes identified a group of 16 miRs that most significantly differentiated them (Table S10). The two histopathological subtypes shared 7 up- and 5 down-regulated plasma miRs. These miRs belonged to those significantly different between BSAll and C (Figure 4).
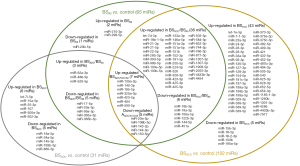
RT-qPCR verification of selected miRs identified in the NGS study
To verify the results obtained using the NGS method, 15, 13, and 10 miRs most strongly associated with the occurrence of NSCLC were selected from the BSAll, BSSCC, BSADC, groups, respectively. RT-qPCR-based measurements in BSAll group of NSCLC patients showed expression changes coherent with the NGS results for 5 out of 8 examined up-regulated miRs and 5 out of 7 examined down-regulated molecules. The RT-qPCR assessments also confirmed significant differences in the expression levels of some of these miRs between the histopathological subtypes of NSCLC, BSSCC and BSADC, with 4 out of 7 up-regulated and 3 out of 6 down-regulated SCC-specific miRs, and 4 out of 5 up-regulated ADC-specific miRs. None of the 5 miRs selected from those demonstrated by NGS as down-regulated in ADC were confirmed by RT-qPCR (Table S11). miR-144-3p, miR-423-5p, miR-451a and miR-590-5p were also identified in a logistic regression model in the context of lung cancer occurrence (Figure 3, Table S6).
The ontological analysis of selected significantly up-regulated miRs in NSCLC patients revealed their involvement in proliferation, cell cycle, and the regulation of metalloendopeptidase activity (Table S12). In the latter process, there were also strongly associated some miRs down-regulated in NSCLC patients. Additionally, these miRs influence apoptosis, cell organisation and differentiation, as well as pulmonary blood vessel remodelling. This group is closely related to important genes (TP53, PTEN, BCL2) and signalling pathways (PI3K AKT, TGFbeta, mTOR) (Table S13).
Pre-surgery plasma miR profiles associated with NSCLC recurrence and overall survival
In NSCLC patients before surgery with (BSRec; n=14) and without (BSNon-rec; n=22) later recurrence, we identified 49 and 33 plasma miRs, respectively, that significantly differentiated patients from controls. These included 41 plasma miRs with higher and 8 miRs with lower levels in BSrec series than in controls (Figure 5, Table S14), and 15 with higher and 18 with lower levels in BSNon-rec series vs. controls (Figure 5, Table S15). Interestingly, we found up-regulation of miR-32-5p in BSRec patients, but in opposite down-regulation of the molecule in BSNon-rec patients.
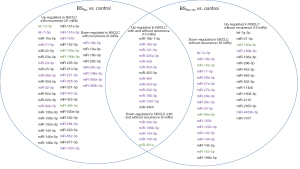
To identify prognostic value of miR levels, multivariable Cox regression analysis was performed, considering DFS and OS. The following variables were included: miR levels, gender, age, histological tumour type, tumour grade, clinical stage. The elevated miR-21-5p and miR-32-5p levels were associated with a higher risk of recurrence, while decreased miR-122b-3p and miR-150-5p levels were associated with OS (Figure S3). Elevated levels of miR-502-3p and miR-92a-3p were linked to both a higher risk of recurrence and reduced OS in NSCLC patients (Figures S4,S5, Table S7). Therefore, the above miRs may independently influence prognosis.
Analysis of the changes in plasma miR levels in NSCLC patients’ paired samples before and after tumour resection
Paired plasma samples collected from NSCLC patients one day before surgery (BSPaired; n=12), and from the same patients at least 1 year (2 years on average) after surgery (ASPaired; n=12) were analysed. The patients showed no clinical recurrence during follow-up (Table S1).
Plasma miR levels before NSCLC resection in a subgroup of patients with plasma miR levels assessed also after tumour resection (BSPaired vs. C)
A 47 plasma miR profile, comprising 6 and 41 miRs increased and decreased, respectively, in BSPaired series vs. controls, differentiated BSPaired subgroup of NSCLC patients before surgery from controls (Table 1, Figure 6). Thirty-six percent of those miRs belonged to the altered plasma miR profiles identified in the whole group of 36 patients (Table S5). A substantial set of 24 molecules associated with recurrences does not show any deregulations in plasma levels in BSPaired series as compared to the control group (up-regulated in BSRec patients: 15a-5p, 22-5p, 23a-3p, 25-3p, 30d-5p, 92a-3p, 92b-3p, 130b-3p, 140-3p, 146a-5p, 148b-3p, 151a-3p, 152-3p, 205-5p, 210-3p, 331-5p, 425-5p, 500a-3p, 502-3p, 629-5p, 877-5p; down-regulated in BSRec patients: 19a-3p, 19b-3p, 29b-3p) (Figure 5).
Table 1
miRs | BaseMean | Log2FC | P value | P adj |
---|---|---|---|---|
Plasma miRs up-regulated in NSCLC patients (6 miRs) | ||||
hsa-miR-1307-5p | 7.25 | 1.27 | 0.009 | 0.08 |
hsa-miR-130a-3p | 45.01 | 0.69 | 0.01 | 0.10 |
hsa-miR-423-5p | 96.77 | 0.49 | 0.03 | 0.19 |
hsa-miR-503-5p | 3.57 | 1.23 | 0.04 | 0.24 |
hsa-miR-133a-3p | 3.74 | 1.44 | 0.049 | 0.24 |
hsa-miR-130a-5p | 0.30 | 2.85 | 0.049 | NA |
Plasma miRs down-regulated in NSCLC patients (41 miRs) | ||||
hsa-miR-6887-5p | 7.88 | −3.78 | <0.001 | <0.001 |
hsa-miR-20b-5p | 20.74 | −1.42 | <0.001 | 0.004 |
hsa-miR-122b-3p | 46.51 | −2.00 | <0.001 | 0.004 |
hsa-miR-93-5p | 141.86 | −0.87 | <0.001 | 0.004 |
hsa-miR-17-5p | 200.82 | −1.15 | <0.001 | 0.004 |
hsa-miR-106a-5p | 35.40 | −1.00 | <0.001 | 0.007 |
hsa-miR-150-5p | 84.49 | −1.42 | <0.001 | 0.01 |
hsa-miR-486-5p | 834.54 | −1.10 | <0.001 | 0.01 |
hsa-miR-222-3p | 11.63 | −1.46 | <0.001 | 0.01 |
hsa-miR-27b-3p | 23.19 | −1.33 | <0.001 | 0.01 |
hsa-miR-125b-5p | 22.48 | −1.44 | <0.001 | 0.01 |
hsa-miR-142-5p | 56.61 | −1.00 | <0.001 | 0.01 |
hsa-miR-181b-5p | 4.37 | −2.07 | 0.001 | 0.02 |
hsa-miR-106b-5p | 319.60 | −0.72 | 0.001 | 0.02 |
hsa-miR-125a-5p | 11.06 | −1.64 | 0.002 | 0.03 |
hsa-miR-15b-3p | 18.00 | −0.96 | 0.002 | 0.04 |
hsa-miR-26b-5p | 84.65 | −0.80 | 0.004 | 0.055 |
hsa-miR-20a-5p | 361.38 | −0.57 | 0.004 | 0.055 |
hsa-miR-126-3p | 142.28 | −0.86 | 0.004 | 0.058 |
hsa-miR-144-3p | 1,091.07 | −0.88 | 0.005 | 0.058 |
hsa-miR-27a-3p | 56.33 | −0.94 | 0.005 | 0.058 |
hsa-miR-30a-5p | 12.30 | −1.05 | 0.005 | 0.06 |
hsa-miR-10b-5p | 12.73 | −1.29 | 0.006 | 0.06 |
hsa-miR-197-3p | 9.04 | −1.36 | 0.007 | 0.06 |
hsa-miR-194-5p | 6.08 | −1.49 | 0.007 | 0.06 |
hsa-miR-99a-5p | 8.29 | −1.42 | 0.007 | 0.06 |
hsa-miR-181a-5p | 37.77 | −1.16 | 0.01 | 0.08 |
hsa-miR-634 | 2.70 | −2.16 | 0.01 | 0.09 |
hsa-miR-192-5p | 13.55 | −0.77 | 0.01 | 0.09 |
hsa-miR-122-5p | 161.52 | −0.99 | 0.01 | 0.10 |
hsa-miR-221-3p | 76.75 | −0.79 | 0.01 | 0.12 |
hsa-miR-26a-5p | 384.20 | −0.88 | 0.01 | 0.13 |
hsa-miR-374b-5p | 7.61 | −1.15 | 0.02 | 0.16 |
hsa-miR-143-3p | 21.32 | −0.99 | 0.02 | 0.18 |
hsa-miR-139-5p | 11.71 | −0.96 | 0.03 | 0.21 |
hsa-miR-18a-5p | 72.97 | −0.51 | 0.03 | 0.21 |
hsa-let-7d-3p | 4.73 | −0.93 | 0.03 | 0.21 |
hsa-miR-32-5p | 8.67 | −0.83 | 0.03 | 0.21 |
hsa-miR-451a | 53,735.61 | −0.37 | 0.04 | 0.24 |
hsa-miR-199a-3p | 121.88 | −0.94 | 0.049 | 0.24 |
hsa-miR-199b-3p | 121.88 | −0.94 | 0.049 | 0.24 |
miRs, microRNAs; FC, fold change; NA, not available; NSCLC, non-small cell lung cancer.
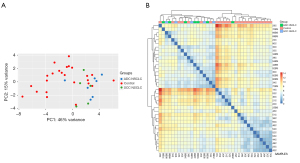
Changes in plasma miR levels in NSCLC patients after tumour resection (BSPaired vs. ASPaired)
Following surgery, plasma levels of 24 miRs increased, while levels of 15 decreased according to the analysis of paired samples from individual patients (Table 2, Figures S6-S8).
Table 2
miRs | Base mean | Log2FC | P value | P adj |
---|---|---|---|---|
Plasma miRs up-regulated in NSCLC patients after surgery (24 miRs) | ||||
hsa-miR-191-5p | 196.39 | 1.13 | <0.001 | 0.007 |
hsa-miR-199a-3p | 132.94 | 1.22 | <0.001 | 0.007 |
hsa-miR-199b-3p | 132.94 | 1.22 | <0.001 | 0.007 |
hsa-miR-21-5p | 421.05 | 1.09 | <0.001 | 0.01 |
hsa-miR-126-5p | 145.33 | 1.29 | 0.001 | 0.02 |
hsa-miR-486-5p | 577.45 | 0.89 | 0.002 | 0.03 |
hsa-miR-181a-5p | 33.96 | 1.31 | 0.002 | 0.03 |
hsa-miR-223-3p | 1,228.53 | 0.98 | 0.002 | 0.03 |
hsa-miR-126-3p | 138.00 | 0.97 | 0.002 | 0.03 |
hsa-miR-221-3p | 87.03 | 1.07 | 0.004 | 0.052 |
hsa-let-7i-5p | 143.96 | 0.59 | 0.005 | 0.052 |
hsa-miR-26a-5p | 310.34 | 0.78 | 0.006 | 0.052 |
hsa-let-7f-5p | 177.08 | 0.63 | 0.007 | 0.052 |
hsa-miR-484 | 210.50 | 0.65 | 0.01 | 0.08 |
hsa-miR-423-3p | 36.31 | 1.01 | 0.01 | 0.08 |
hsa-miR-151a-5p | 81.76 | 0.63 | 0.01 | 0.09 |
hsa-miR-197-3p | 9.93 | 1.42 | 0.01 | 0.10 |
hsa-miR-24-3p | 232.86 | 0.75 | 0.01 | 0.10 |
hsa-miR-150-5p | 59.08 | 0.82 | 0.02 | 0.14 |
hsa-miR-744-5p | 10.08 | 1.37 | 0.02 | 0.14 |
hsa-miR-151b | 94.94 | 0.54 | 0.03 | 0.16 |
hsa-miR-4286 | 33.08 | 0.97 | 0.04 | 0.18 |
hsa-miR-181b-5p | 3.53 | 1.60 | 0.04 | NA |
hsa-let-7g-5p | 205.82 | 0.46 | 0.047 | 0.20 |
Plasma miRs down-regulated in NSCLC patients after surgery (15 miRs) | ||||
hsa-miR-15b-5p | 264.76 | −1.41 | <0.001 | 0.007 |
hsa-miR-122b-3p | 8.98 | −2.08 | <0.001 | 0.01 |
hsa-miR-29c-3p | 15.38 | −1.27 | 0.005 | 0.052 |
hsa-miR-144-3p | 552.85 | −0.74 | 0.006 | 0.052 |
hsa-miR-7-5p | 66.76 | −1.01 | 0.006 | 0.052 |
hsa-miR-450a-5p | 2.18 | −2.36 | 0.009 | NA |
hsa-miR-205-5p | 5.99 | −1.48 | 0.01 | 0.08 |
hsa-miR-369-3p | 1.76 | −1.77 | 0.02 | NA |
hsa-miR-106b-5p | 176.76 | −0.52 | 0.03 | 0.14 |
hsa-miR-885-5p | 2.18 | −1.92 | 0.03 | NA |
hsa-miR-365a-3p | 2.74 | −1.57 | 0.03 | NA |
hsa-miR-365b-3p | 2.74 | −1.57 | 0.03 | NA |
hsa-miR-101-5p | 1.53 | −1.69 | 0.03 | NA |
hsa-miR-219a-5p | 0.61 | −3.73 | 0.04 | NA |
hsa-miR-22-3p | 127.77 | −0.46 | 0.04 | 0.20 |
miRs, microRNAs; FC, fold change; NA, not available; NSCLC, non-small cell lung cancer.
Plasma miR levels in NSCLC patients after tumour resection compared to normal levels (ASPaired vs. C)
In the ASPaired samples from NSCLC patients, the levels of 51 plasma miRs were higher and of 51 were lower, compared to that in controls (Table 3, Figure 7). Some of these miRs were also identified in the plasma of BSRec patients with recurrences (up-regulated miRs: 7i-5p, 21-5p, 24-3p, 26b-3p, 99b-5p, 151a-5p, 151b, 221-3p, 328-3p, 423-3p, 584-5p, 652-3p, 1307-3p and down-regulated miRs: 18b-5p, 29c-3p, 106a-5p, 365a-3p, 365b-3p) (Figure 5).
Table 3
miRs | Base mean | Log2FC | P value | P adj |
---|---|---|---|---|
Plasma miRs up-regulated in NSCLC patients after surgery (51 miRs) | ||||
hsa-miR-191-5p | 249.49 | 1.50 | <0.001 | <0.001 |
hsa-miR-130a-3p | 77.32 | 0.98 | <0.001 | <0.001 |
hsa-miR-423-5p | 146.98 | 0.80 | <0.001 | <0.001 |
hsa-miR-423-3p | 50.24 | 1.37 | <0.001 | <0.001 |
hsa-miR-151a-5p | 121.29 | 0.81 | <0.001 | <0.001 |
hsa-miR-151b | 142.68 | 0.71 | <0.001 | <0.001 |
hsa-miR-484 | 305.00 | 0.79 | <0.001 | <0.001 |
hsa-miR-744-5p | 13.28 | 1.59 | <0.001 | <0.001 |
hsa-let-7f-5p | 263.21 | 0.71 | <0.001 | <0.001 |
hsa-miR-33a-5p | 15.00 | 1.16 | <0.001 | 0.001 |
hsa-let-7i-5p | 233.98 | 0.61 | <0.001 | 0.001 |
hsa-let-7a-5p | 467.04 | 0.58 | <0.001 | 0.001 |
hsa-miR-326 | 18.15 | 1.16 | <0.001 | 0.002 |
hsa-miR-24-3p | 344.51 | 0.83 | <0.001 | 0.006 |
hsa-miR-652-3p | 31.44 | 0.77 | <0.001 | 0.007 |
hsa-miR-766-3p | 7.12 | 1.18 | 0.001 | 0.01 |
hsa-miR-21-5p | 669.96 | 0.76 | 0.001 | 0.01 |
hsa-miR-130b-5p | 2.23 | 1.31 | 0.001 | 0.01 |
hsa-miR-126-5p | 241.67 | 0.75 | 0.001 | 0.01 |
hsa-miR-185-5p | 427.49 | 0.50 | 0.001 | 0.01 |
hsa-miR-1307-5p | 10.67 | 0.97 | 0.001 | 0.01 |
hsa-miR-1307-3p | 9.01 | 0.94 | 0.001 | 0.01 |
hsa-miR-133a-3p | 6.23 | 1.48 | 0.002 | 0.02 |
hsa-miR-1260b | 30.28 | 0.96 | 0.004 | 0.02 |
hsa-miR-320a-3p | 158.73 | 0.64 | 0.004 | 0.03 |
hsa-miR-223-3p | 1,863.12 | 0.78 | 0.005 | 0.03 |
hsa-miR-221-3p | 152.49 | 0.71 | 0.005 | 0.03 |
hsa-miR-584-5p | 11.28 | 1.06 | 0.005 | 0.03 |
hsa-miR-4286 | 51.03 | 0.91 | 0.005 | 0.03 |
hsa-miR-4433b-3p | 0.99 | 1.80 | 0.006 | 0.04 |
hsa-let-7c-5p | 5.87 | 0.83 | 0.009 | 0.054 |
hsa-miR-99b-5p | 3.28 | 1.15 | 0.01 | 0.06 |
hsa-miR-128-3p | 57.55 | 0.44 | 0.01 | 0.07 |
hsa-let-7d-5p | 52.82 | 0.48 | 0.01 | 0.07 |
hsa-miR-7-1-3p | 2.34 | 1.35 | 0.01 | 0.07 |
hsa-miR-197-3p | 19.59 | 0.85 | 0.01 | 0.07 |
hsa-let-7b-5p | 307.71 | 0.44 | 0.01 | 0.08 |
hsa-miR-186-5p | 41.29 | 0.41 | 0.01 | 0.09 |
hsa-miR-4433b-5p | 30.12 | 0.95 | 0.02 | 0.09 |
hsa-miR-590-5p | 11.63 | 0.59 | 0.02 | 0.09 |
hsa-miR-486-3p | 9.23 | 0.68 | 0.02 | 0.09 |
hsa-miR-376c-3p | 33.60 | 0.80 | 0.02 | 0.09 |
hsa-miR-625-3p | 4.52 | 1.05 | 0.02 | 0.11 |
hsa-miR-26b-3p | 1.75 | 0.92 | 0.02 | 0.11 |
hsa-miR-328-3p | 2.31 | 1.10 | 0.02 | 0.12 |
hsa-let-7g-5p | 340.57 | 0.29 | 0.03 | 0.15 |
hsa-miR-339-5p | 38.46 | 0.50 | 0.03 | 0.15 |
hsa-miR-199a-5p | 20.16 | 0.76 | 0.03 | 0.15 |
hsa-miR-503-5p | 3.42 | 0.95 | 0.04 | 0.17 |
hsa-miR-376b-3p | 5.79 | 0.84 | 0.049 | 0.19 |
hsa-miR-98-5p | 12.04 | 0.42 | 0.049 | 0.19 |
Plasma miRs down-regulated in NSCLC patients after surgery (51 miRs) | ||||
hsa-miR-122-5p | 210.56 | −1.98 | <0.001 | <0.001 |
hsa-miR-30a-5p | 16.54 | −1.51 | <0.001 | <0.001 |
hsa-miR-122b-3p | 68.32 | −3.42 | <0.001 | <0.001 |
hsa-miR-194-5p | 8.71 | −2.21 | <0.001 | <0.001 |
hsa-miR-106b-5p | 457.90 | −1.22 | <0.001 | <0.001 |
hsa-miR-27b-3p | 32.57 | −1.28 | <0.001 | <0.001 |
hsa-miR-103b | 2.21 | −4.58 | <0.001 | <0.001 |
hsa-miR-365a-3p | 6.45 | −1.70 | <0.001 | <0.001 |
hsa-miR-365b-3p | 6.45 | −1.70 | <0.001 | <0.001 |
hsa-miR-143-3p | 29.41 | −1.60 | <0.001 | <0.001 |
hsa-miR-32-5p | 13.79 | −1.15 | <0.001 | <0.001 |
hsa-miR-125b-5p | 32.72 | −1.22 | <0.001 | <0.001 |
hsa-miR-27a-3p | 85.02 | −0.87 | <0.001 | <0.001 |
hsa-miR-375-3p | 2.80 | −2.60 | <0.001 | 0.001 |
hsa-miR-106a-5p | 54.24 | −0.83 | <0.001 | 0.003 |
hsa-miR-144-3p | 1,686.92 | −1.38 | <0.001 | 0.004 |
hsa-miR-20b-5p | 34.82 | −0.96 | <0.001 | 0.004 |
hsa-miR-363-3p | 19.53 | −0.75 | <0.001 | 0.004 |
hsa-miR-192-5p | 21.40 | −0.65 | <0.001 | 0.006 |
hsa-miR-214-3p | 2.30 | −1.74 | <0.001 | 0.01 |
hsa-miR-30e-5p | 116.58 | −0.51 | 0.001 | 0.01 |
hsa-miR-144-5p | 84.71 | −0.77 | 0.002 | 0.01 |
hsa-miR-20a-5p | 560.94 | −0.50 | 0.002 | 0.02 |
hsa-miR-6887-5p | 12.45 | −1.68 | 0.003 | 0.02 |
hsa-miR-7-5p | 116.11 | −0.76 | 0.003 | 0.02 |
hsa-miR-93-5p | 230.53 | −0.53 | 0.003 | 0.02 |
hsa-miR-16-5p | 2,978.59 | −0.49 | 0.003 | 0.02 |
hsa-miR-634 | 4.47 | −1.71 | 0.003 | 0.02 |
hsa-miR-17-5p | 323.07 | −0.74 | 0.004 | 0.02 |
hsa-miR-205-5p | 10.86 | −0.99 | 0.004 | 0.02 |
hsa-miR-30b-5p | 64.23 | −0.54 | 0.004 | 0.03 |
hsa-miR-15b-5p | 519.64 | −1.04 | 0.005 | 0.03 |
hsa-miR-30c-5p | 160.81 | −0.54 | 0.006 | 0.04 |
hsa-miR-374a-5p | 1.76 | −1.34 | 0.006 | 0.04 |
hsa-miR-340-5p | 18.68 | −0.74 | 0.007 | 0.04 |
hsa-miR-145-3p | 0.76 | −1.82 | 0.007 | 0.04 |
hsa-let-7a-3p | 14.32 | −0.88 | 0.01 | 0.05 |
hsa-miR-18a-3p | 0.41 | −2.32 | 0.01 | 0.06 |
hsa-miR-10b-5p | 19.78 | −0.85 | 0.01 | 0.07 |
hsa-miR-29a-3p | 78.11 | −0.67 | 0.01 | 0.07 |
hsa-miR-219b-3p | 0.45 | −2.91 | 0.01 | 0.07 |
hsa-miR-18b-5p | 17.74 | −0.69 | 0.01 | 0.07 |
hsa-miR-143-5p | 1.79 | −1.91 | 0.01 | 0.08 |
hsa-miR-885-5p | 3.46 | −1.20 | 0.01 | 0.09 |
hsa-miR-26b-5p | 133.84 | −0.46 | 0.01 | 0.09 |
hsa-miR-29c-3p | 29.68 | −0.85 | 0.02 | 0.09 |
hsa-miR-9985 | 0.94 | −1.64 | 0.02 | 0.09 |
hsa-miR-16-1-3p | 0.29 | −1.88 | 0.02 | NA |
hsa-miR-142-5p | 84.18 | −0.40 | 0.03 | 0.13 |
hsa-miR-125b-2-3p | 0.45 | −2.09 | 0.03 | 0.15 |
hsa-miR-5010-3p | 0.48 | −1.80 | 0.04 | 0.17 |
miRs, microRNAs; FC, fold change; NA, not available; NSCLC, non-small cell lung cancer.
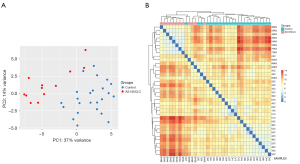
Pre-surgery plasma miR alterations that normalized after NSCLC resection
After tumour resection, one of six miRs with increased pre-surgery plasma levels (miR-130a-5p), and 17 out of the 41 miRs with decreased pre-surgery plasma levels in BSPaired subgroup returned to the levels comparable to those in controls (normalised) (Figure 8). However, based on the analyses comprising paired samples, out of the 17 mentioned above, 9 miRs increased in individual patients (BSPaired vs. ASPaired) (Table 2, Figure 7, Figure S6A).
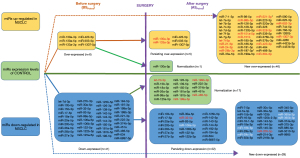
The functional analyses showed that the miRs whose levels normalised after surgery (n=18) are specific to blood, predominantly to leukocytes. They are associated with many important carcinogenesis-related biological, immunological, inflammatory processes. They are involved in cellular responses to stress, injury, hypoxia (FoxO, HIF-1 pathways), and cigarette smoking (AhR receptors). The genes most strongly associated with these miRs play critical roles in proliferation, inhibition of apoptosis, angiogenesis, and cell migration. Notable genes include BCL2, AKT1, MTOR, PTEN, MEG3, and SSX2IP. Important terms acquired in functional analysis of this set of miRs are summarised in Table 4, full list is available in supplementary materials (table available at https://cdn.amegroups.cn/static/public/tlcr-24-626-1.xlsx).
Table 4
Category | Important ontological terms (q-value) |
---|---|
Cell/tissue specific | Blood |
Leukocyte | |
Carcinogenesis | Proteoglicans in cancer |
Cancer pathways | |
Cell death | Autophagia/macroautophagia |
Apoptosis | |
Anoikis | |
Cell proliferation | Proliferation |
Cell cycle | |
Epithelial cell proliferation | |
Cell plasticity | Regulation of epithelial-mesenchymal transition (EMT) |
Inter-cells contact/cell migration/motility | Cell migration/motility |
Focal adhesion | |
Negative regulation of metallopeptidase activity | |
Response to external factors | Cellular response to stress |
Cigarette smoking [e.g., aryl hydrocarbon receptor (AhR)] | |
DNA damage response | |
Hipoxia (FoxO, HIF-1 pathways) | |
Response to wounding | |
Senescence/oxidative stress | |
Starvation | |
Blood cells | B-cell receptor sig. path/B-cell proliferation/differentiation |
T cell receptor sig. path/T cell death/differentiation/proliferation | |
Hemapoetic stem cell gene regulation by GABP complex | |
Leukocyte transendothelial migration | |
Mitochondria | Copper homeostasis |
Mitochondrial depolarization | |
Mitochondrial membrane potential | |
Protein localization to mitochondria | |
Release of cytochrome c from mitochondria during apoptosis | |
Immune system response | Chemokine |
Inflammantion/infection/prostaglandin | |
Interleukin | |
Regulation of immune system response, process/development | |
Response to cytokine/cytokine signaling | |
Tumor necrosis factor (TNF) | |
Metabolism | Adipocite signaling path/adipogenesis |
Carbohydrate digestion and absorption | |
Glucose metabolic process | |
Lipid metabolism/lipolysis in adipocytes | |
Metabolism of RNA | |
Nitrogen compound metabolic process | |
Nitric oxide synthases (NOS) regulator activity | |
Protein metabolic process/modification/amino acids | |
Blood vessels | Angiogenesis |
Endothelial cell proliferation/angiogenesis | |
Neovascularization | |
Bones and cartilage tissue | Bone morphogenesis |
Endochondral ossification | |
Osteoclast differentiation | |
Nervous system | Negative regulation of neuron projection development |
Nervous system | |
Neurogenesis | |
Heart | Positive regulation of cardiac muscle cell proliferation |
Cardiac hypertrophic response | |
Adrenergic signaling in cardiomyocytes | |
Growth factor/growth factor receptors | Insulin-like growth factor/1 receptor (IGF/1R) |
Nerve growth factor (NGF) | |
Transforming growth factor β (TGFβ) | |
Vascular endothelial growth factor (VEGF) | |
Hormones | Estrogene |
Insulin | |
Thyroid hormone signaling pathway/thyroid stimulating hormone (TSH) | |
Notable genes | BCL2 |
AKT1 | |
MTOR | |
PTEN | |
MEG3 | |
SSX2IP | |
Signalling pathways | MAPK |
PI3K/AKT1 | |
mTOR | |
TP 53 network |
Some of NSCLC-associated plasma miR alterations persisted after surgery
In the ASPaired samples from patients without recurrence, some of the NSCLC-associated plasma miR changes observed before surgery persisted despite tumour resection. These included 5 out of all the 6 miRs (83%) that were overexpressed before surgery, and 22 out of all the 41 pre-surgery down-regulated miRs (53.7%) (Figure 8).
The functional analysis showed that NSCLC-associated plasma miRs (n=27) whose alterations persisted after surgery are predominantly liked to tissues such as blood vessels, lung, and pleura, with additional sources including myocardium, bladder, prostate, and spinal cord. These miRs are associated with various processes, in lung tissue as well as lung diseases [chronic obstructive pulmonary disease (COPD), lung cancer, NSCLC, fibrosis], processes occurring in bones and cartilage and the functioning of the heart and its blood vessels. They take part in the immune system processes and development, activity of platelets (platelet dense granule organization/activation), hypoxia, inflammation, wound healing. In this group, there are miRs associated with mitochondrial activity involved in the apoptotic processes [activation of Phorbol-12-myristate-13-acetate-induced protein 1 (Noxa) and translocation to mitochondria]. The genes these miRs are most closely associated with are responsible for the following processes: cell proliferation and differentiation (HOXD11, MAPK9, UBR5), histone modification (KMT5B) and circulation and blood pressure in the lungs (BMPR2). Important terms identified in functional analysis are summarised in Table 5, the full list is available in supplementary materials (table available at https://cdn.amegroups.cn/static/public/tlcr-24-626-2.xlsx)
Table 5
Category | Ontological terms (q-value) |
---|---|
Cell/tissue specific | Artery |
Bladder | |
Brain/arachnoid mater | |
Lung | |
Lymph node | |
Myocardium | |
Muscle | |
Nerve | |
Pleura | |
Prostate | |
Spinal cord | |
Vein | |
Carcinogenesis | Proteoglicans in cancer |
Cancer pathways | |
Cell death | Autophagia/macroautophagia |
Apoptosis | |
Anoikis | |
Cell proliferation | Proliferation |
Neuroblast proliferation | |
Cell differentiation/maturation | |
Cell cycle | |
Cell plasticity | Positive regulation of epithelial-mesenchymal transition (EMT) |
Inter-cells contact/cell migration/motility | Cell matrix adhesion/cell substrate adhesion |
Cell migration/motility | |
Extracellular matrix receptor (ECM-receptor) | |
Focal adhesion | |
Chromatin organisation | Histone modification/acetylation |
Response to external factors | Cellular response to stress |
DNA damage response | |
Hipoxia | |
Negative regulation of connective tissue replacement involved in inflammatory response wound healing | |
Senescence/oxidative stress | |
Starvation | |
Blood cells | B-cell/T-cell proliferation/differentiation |
Hematopoietic cell lineage | |
Hemapoetic stem cell gene regulation by GABP complex | |
Leukocyte transendothelial migration | |
Monocyte differentiation | |
Platelet degranulation | |
Platelet dense granule organization/activation | |
Mitochondria | Activation of NOXA and translocation to mitochondria |
Copper homeostasis | |
Mitochondrial membrane potential/protein localization to mitochondria | |
Mitochondrial premeability transition pore complex (MPTP complex) | |
Positive regulation of parkin-mediated stimulation of mitophagy in response to mitochondrial depolarization/mitophagy | |
Immune response | Chemokine |
Inflammantion/infection/prostaglandin | |
Interleukins | |
Modulation by host of viral process regulation of immune system response | |
Regulation of immune system response process/development | |
Response to cytokine/cytokine signaling | |
Metabolic processes | Lipid metabolism/biosynthetic/lipolysis in adipocytes |
Negative regulation of lipid storage/lipid binding | |
Positive regulation of low-density lipoprotein receptor activity | |
Positive regulation of high-density lipoprotein particle assembly | |
Adipocite signaling path/adipogenesis | |
Biosynthetic process | |
Catabolic process | |
Cholesterole transport/apolipoprotein A-I receptor activity | |
Protein metabolic process/modification/amino acids | |
Nitrogen compound metabolic process | |
Blood vessels | Endothelial cell proliferation/angiogenesis |
Vascular associated smooth muscle cell proliferation (VSMC) | |
Bones and cartilage tissue | Bone morphogenesis |
Chondrocyte proliferation/development | |
Endochondral ossification | |
Negative regulation of osteoblast differentiation | |
Osteoclast differentiation | |
Heart | Cardiac myofibryl asembly |
Cardiac muscle hipertrophy | |
Cardiovascular disease | |
Coronary artery morphogenesis | |
Dilated cardiomyopathy | |
Endocardial cell differentiation | |
Heart development | |
Heart morphogenesis | |
Left ventricular cardiac muscle tissue morphogenesis | |
microRNAs in cardiomyocyte hypertrophy | |
Pathogeniesis of cardiovascular diseases | |
Regulation of cell proliferation involved in heart valve morphogenesis | |
Semi-lunar valve development | |
Lung | Chronic obstructive pulmonary disease (COPD) |
Lung blood pressure | |
Lung alveolus development | |
Positive regulation of pulmonary blood vessel remodeling | |
Nervous system | Nervous system development |
Growth factor | Epidermal growth factor receptor (EGFR) |
Insulin-like growth factor/1 receptor (IGF/1R) | |
Platelet-derived growth factor (PDGF) | |
Transforming growth factor β (TGFβ) | |
Vascular endothelial growth factor (VEGF) | |
Hormones | Androgene/receptor signaling pathway |
Estrogene | |
Insulin | |
Prolactin signaling pathway | |
Notable genes | HOXD11 |
MAPK9 | |
UBR5 | |
BMPR2 | |
KMT5B | |
Selected signalling pathways | ATM dependent DNA damage/damage response |
BMP | |
DDX58IFIH1 | |
ERB 2 | |
Embryonic stem cells (ESC) pluripotency and self-renewal signaling pathway | |
NIKNF-kappa B | |
PI3K/AKT1 | |
PTEN | |
RUNX3 | |
SMAD23 | |
SMAD/SMAD2SMAD3SMAD4 | |
mTOR | |
TP 53 network |
Novel plasma miR alterations in NSCLC patients after tumour resection
Forty six out of 51 (90%) miRs with increased, and 29 out of 51 (57%) with decreased plasma levels after surgery, exhibited similar levels to those in blood donors without cancer before surgery. Additionally, plasma levels of miR-221-3p and miR-197-3p, found to be significantly lower in untreated NSCLC patients than in normal donors, increased after surgery and significantly exceeded normal levels (Figure 8, Figure S6B).
Functional analysis showed that the miRs presenting novel alterations in plasma levels after tumour resection (n=75) were sperm-, neuroepithelial stem cell-, mesenchymal stromal cell (MSC)- and myotube-specific. They were associated with many critical signalling pathways responsible for cell proliferation and differentiation. Furthermore, they play roles in epigenetic processes, such as remodelling and organisation of chromatin (SWI/SNF family chromatin remodeling complexes), nucleosome organisation, histone modification, and in the functioning of stem cells, including somatic stem cell population maintenance, pluripotency of stem cell. In addition, these miRs influence different cell death mechanisms. They are related to mitochondrial activity and activation of p53 up-regulated modulator of apoptosis (PUMA) pathway. This set of miRs is also involved in the immune system processes and is associated with the formation and development of cardiac vascular smooth muscle cell, coronary vain morphogenesis and cardiovascular disease. miRs associated with platelet formation were also included. The genes most strongly regulated by these miRs are responsible for mRNA degradation processes (EDC3), Nonsense Mediated Decay NMD independent of the Exon Junction Complex (EJC)-post-transcriptional gene regulation (EEF1A1), apoptosis (CASP3) and cell proliferation (MYC). Important terms identified in functional analysis are summarised in Table 6, the full list is available in supplementary materials (table available at https://cdn.amegroups.cn/static/public/tlcr-24-626-3.xlsx).
Table 6
Category | Ontological terms (q-value) |
---|---|
Cell/tissue specific | Leukocyte |
Neuroepithelial stem cell | |
Mesenchymal stromal cell (MSC) | |
Myotube specific | |
Sperm specific | |
Carcinogenesis | Proteoglicans in cancer |
Cancer pathways | |
Cell death | Autophagia/macroautophagia |
Apoptosis | |
Anoikis | |
Pyroptosis | |
Necroptotic process | |
Ferroptosis | |
Fibroblast apoptotic process | |
Cell proliferation | Cell cycle |
Cell plasticity | Positive regulation of epithelial-mesenchymal transition (EMT) |
Somatic stem cell population maintenance | |
Pluripotency of stem cell | |
Inter-cell contact/cell migration/motility | Regulation of stress fiber assembly |
Focal adhesion | |
Cell matrix adhesion/cell substrate adhesion | |
Chromatin organisation | Histone modification |
Nucleosome organization | |
Pericentric/subtelomeric heterochromatin formation | |
Remodeling and organization chromatin (SWI/SNF complex) | |
Response to external factors | Cellular response to stress |
Starvation | |
DNA damage response | |
Senescence/oxidative stress | |
Blood cells | B-cell proliferation/differentiation |
T cell death/differentiation/proliferation | |
Leukocyte transendothelial migration | |
Macrophage differentiation | |
Platelet formation | |
Mitochondria | Activation of p53 upregulated modulator of apoptosis (PUMA) and translocation to mitochondria |
Mitochondrial activity (rRNA/tRNA in mitochondrion) | |
Positive regulation of parkin-mediated stimulation of mitophagy in response to mitochondrial depolarization/mitophagy | |
Immune response | Inflammantion/infection |
Interleukins (IL2, IL3, IL4, IL5, IL10, IL13) | |
Modulation by host of viral process regulation of immune system response | |
Response to cytokine/cytokine signaling | |
Tumor necrosis factor (TNF) | |
Translational silencing of ceruloplasmin expression | |
Virus infection/viral process | |
Metabolism | Adipocite signaling path/adipogenesis |
Biosynthetic process | |
Catabolic process | |
Fatty acid synthase activity/biosynthesis/degradation | |
Protein metabolic process/modification/amino acids | |
Nitrogen compound metabolic process | |
Blood vessels | Angiogenesis |
Blood vessel endothelial cell migration | |
Heart | Arrhythmogenic right ventricular cardiomyopathy (ARVC) |
Cardiac vascular smooth muscle cell development | |
Cardiovascular disease | |
Coronary artery morphogenesis | |
Coronary vain morphogenesis | |
Heart morphogenesis | |
Nervous system | Negative regulation of neuron projection development |
Nervous system | |
Neurogenesis | |
Growth factor/growth factor receptors | Insulin-like growth factor/1 receptor (IGF/1R) |
Transforming growth factor β (TGFβ) | |
Vascular endothelial growth factor (VEGF) | |
Hormones | Cortisol synthesis and secretion |
Insulin | |
Prolactin signaling pathway | |
Thyroid hormone signaling pathway/thyroid stimulating hormone (TSH) | |
Notable genes | EDC3 |
CASP3 | |
EEF1A1 | |
MYC | |
Selected signalling pathways | FoxO signaling pathway |
TP 53 network | |
Hippo-Merlin | |
Yap/TAZ | |
ROBO/SLITs | |
Longevity signaling pathway | |
Wnt (pluripotency and self-renewal signaling pathway)/Wnt/B-catenin | |
SWI/SNF complex |
Discussion
Untreated patients with NSCLC exhibited notable changes in plasma miR levels compared to cancer-free donors. A subset of those miRs was found to be associated with recurrence. Interestingly, even after at least one year post NSCLC resection, patients who showed no clinical evidence of recurrence retained some of the pre-surgery changes while also manifesting new changes.
Alterations in plasma miR levels may reflect various tumour biology mechanisms. Plasma miRs in NSCLC patients can originate not only directly from primary tumours, circulating cancer cells, dormant micrometastases, and pre-cancer cells in distant organs but also from stimulated neighbouring tissues. This has been substantiated by multiple studies.
First, a substantial body of evidence indicates that the dissemination of cancer cells, including NSCLC cells, can occur early (21,22). In 60% of nodule-negative NSCLC patients without clinical evidence of distant metastases, haematogenous dissemination of cancer cells was demonstrated (23). The early disseminated cancer cells can colonise various organs and persist for many years as dormant cells in patients without any clinical features of metastasis (24). Furthermore, epithelial non-transformed cells have been suggested to disseminate and colonise various organs before the onset of malignant transformation, thereby contributing to metastasis (25). Circulating tumour cells with clear malignant features have been found in patients with chronic obstructive pulmonary disease, a risk factor for lung cancer (26). In patients undergoing surgery for benign pulmonary diseases Nordgård et al. detected microscopic NSCLC metastases in control lymph nodes (27).
Second, pre-surgery plasma miR alterations may represent a systemic response to cancer, involving the phenomenon of tumour education of cells from other tissues, including platelets and other blood cells (28). For example, whole blood cells from NSCLC patients have been shown to present deregulated miR expression (29,30).
In NSCLC patients, the levels of some of the pre-surgery deregulated plasma miRs returned to levels comparable to those in cancer-free donors after tumour resection. The post-surgery normalisation suggests that the abnormal levels of those plasma miRs were influenced by the presence of the primary tumour and may have potential as a marker. This is in line with the understanding of the tumour as an abnormal tissue interacting with the entire organism (31), and with previous observations in patients with gastric, hepatocellular, and breast carcinoma, where increased pre-operative levels of some plasma miRs returned to a normal levels after tumour resection (32-34).
This study revealed a set of pre-surgery plasma miRs associated with recurrence, which showed altered levels compared to thoset in cancer-free donors. In addition, some miRs were shown to have independent prognostic value. These included miR-21-5p, miR-32-5p miR-122b-3p, miR-150-5p, miR-502-3p, and miR-92a-3p. Interestingly, pre-surgery plasma miR-32-5p was up-regulated in patients with recurrence, while it was down-regulated in those without recurrence. It is worth noting that a recent study has suggested a tumour suppressor role for miR-32-5p in NSCLC (35).
We found some recurrence-associated alterations both in plasma of BSPaired as well in ASPaired patients without recurrence during long-term follow-up (from 4 to 12 years after surgery). Approximately 90% of NSCLC recurrences develop within the first 2–3 years after curative resection (36). Additionally, NSCLC patients are at a higher risk for developing a second cancer (37). Noteworthy, in long-term monitoring of patients without clinical evidence of recurrence at the second collection of plasma samples, four patients developed a second primary malignancy, including: two patients with NSCLC (ADC) and two patients with prostate cancer. It is possible that these recurrence-associated alterations in the plasma of BSPaired patients reflect some systemic changes or pre-cancer effects.
The presented study also showed that the majority of the pre-surgery plasma miR changes persisted despite tumour removal and the absence of clinical evidence of recurrence. The persisted plasma miRs alterations can be associated with the occult/dormant lymph node metastases (23,27) and/or the disseminated non-cancer/pre-cancer cells that can secrete miRs and/or stimulate secretion by neighbouring tissues (25).
Additionally, since all post-surgery-examined patients were smokers, smoking-related plasma miRs may have contributed to some of the plasma miR level changes persisting after surgery. Noteworthy, half of the miRs exhibiting persisted plasma level changes have been previously linked to smoking (38,39), and four of those miRs (miR-10b-5p, miR-106b-5p, miR-106a-5p and miR-125b-5p) have been found to be differentially expressed in bronchial epithelium in smokers and non-smokers (40).
The alterations persisting after tumour removal, may represent “field cancerisation” or “field of injury” phenomena (41), perhaps associated with smoking. A context of “tissue organization field theory” (TOFT), perceiving cancer as a tissue-based disease that arises following the disruption of reciprocal interactions between parenchyma and stroma resulting in altered tissue organisation, may also be considered (42). Finally, since histologically normal lung tissue from lung cancer patients exhibits a progression-associated inflammatory gene signature (43), activation of inflammatory pathways in the NSCLC macroenvironment may have also contributed to the permanently altered plasma miR profile described here.
Post-surgery profiling revealed novel plasma miR alterations not observed before surgery, differentiating patients from cancer-free donors. This phenomenon may be linked to several factors. Cancer surgery, including that in NSCLC patients, on the one hand has been shown to generate an increased number of circulating cancer cells (44) that can convert into dormant micrometastases in distant organs. On the other hand, it has been shown to modulate tumour dormancy (45). Dormant micrometastases, as already mentioned above, can contribute to the observed new plasma miR profiles. Another mechanism linked to lung cancer resection that contributes to new plasma miR changes, may be related to systemic postoperative hypoxemia resulting from the partial removal of a significant portion (20–50%) of lung tissue (46). The removal can lead to systemic oxygen deficiency—hypoxemia—resulting in reduced oxygenation of various tissues (hypoxia), with further changes relating to biogenesis of miRs being influenced by hypoxia. It has been demonstrated that hypoxia can induce or downregulate the expression of various miRs (47). We observed that the group of miRs demonstrating new post-surgery alterations included 6 overexpressed miRs (miR-21-5p, let7-b-5p, let-7a-5p, miR-98-5p, let-7c-5p and let-7i-5p), whose expression is upregulated by hypoxia. In addition, the new post-surgery alterations included three overexpressed miRs, whose expression is downregulated by hypoxia (miR-17b-5p, miR-16-5p and miR144-5p).
The observed novel post-surgery plasma miR profiles were also associated with stem cells, megakaryocytes and platelets, and may reflect some systemic changes after full or partial lobectomy during lung cancer surgery. This is in line with the suggested physiological role of lung megakaryocytes in thrombopoiesis, as well as with the discovery of abundant megakaryocytes found in pulmonary artery blood in lung cancer patients (48,49).
We found that terms related to mitochondria and their participation in apoptosis also appeared in the functional analysis of the novel post-surgery plasma miR alterations. Thus, new post-surgery alterations in mitochondrial function-associated plasma miRs (mitochondrial activity, rRNA/tRNA in mitochondrion) may reflect a post-surgery systemic change linked to mitochondria transfer (50).
The strength of our study is that for the first time the analysis of changes in plasma miRs profiles was performed in carefully defined group of NSCLC patients showing no clinical evidence of recurrence during long-term follow-up (from 4 to 12 years) after surgery. In addition, for the first time it was found that in NSCLC patients after tumour excision, some circulating miR profile changes persist and novel miR changes emerge despite the absence of clinical symptoms of recurrence. One of the limitations of our study is the small size of the patients’ cohort. However, the statistical power of our tests was sufficient to identify potential biomarkers of significant relevance, and our preliminary analyses showed some promising diagnostic/prognostic associations, but the confirmation of their application potential will require further studies including a larger cohort of patients. Another limitation is the lack of analysis of the observed plasma miR profiles in the context of environmental exposures.
Conclusions
In summary, the study provides new insights into the dynamics of plasma miR alterations following NSCLC resection. The pre-surgery plasma miR profile in NSCLC patients significantly differs from that of normal blood donors. It encompasses recurrence-associated set of miRs, including miR-21-5p, miR-32-5p miR-122b-3p, miR-150-5p, miR-502-3p, and miR-92a-3p which show independent prognostic value. Furthermore, miR-32-5p exhibited notable opposite changes in patients with and without recurrence, what additionally suggesting its biomarker potential. Noteworthy, we demonstrated for the first time that in NSCLC patients with no clinical evidence of recurrence during long-term follow-up after tumour resection (from 4 to 12 years after surgery) some pre-surgery alterations normalised, novel changes emerged, but the majority of the pre-surgery alterations persisted. The long-term persistence of the NSCLC-related plasma miRs abnormalities despite tumour resection may indicate the existence of permanent systemic alterations that predispose or are induced by lung cancer. The observations provide further support of the postulated (51) development of new therapeutic strategies targeting both cancer cells and the tumour macroenvironment.
Acknowledgments
This work was supported by Jakub hr. Potocki Foundation, Warsaw, Poland.
Footnote
Reporting Checklist: The authors have completed the MDAR reporting checklist. Available at https://tlcr.amegroups.com/article/view/10.21037/tlcr-24-626/rc
Data Sharing Statement: Available at https://tlcr.amegroups.com/article/view/10.21037/tlcr-24-626/dss
Peer Review File: Available at https://tlcr.amegroups.com/article/view/10.21037/tlcr-24-626/prf
Funding: This work was supported by
Conflicts of Interest: All authors have completed the ICMJE uniform disclosure form (available at https://tlcr.amegroups.com/article/view/10.21037/tlcr-24-626/coif). J.K.S. reports funding support from Jakub hr. Potocki Foundation (agreement number 658/19). The other authors have no conflicts of interest to declare.
Ethical Statement: The authors are accountable for all aspects of the work in ensuring that questions related to the accuracy or integrity of any part of the work are appropriately investigated and resolved. The study was conducted in accordance with the Declaration of Helsinki (as revised in 2013). The study was approved by Bioethics Committee of Maria Sklodowska-Curie National Research Institute of Oncology in Warsaw (No. 4/2011/1/2012), and written informed consent was obtained from sample donors.
Open Access Statement: This is an Open Access article distributed in accordance with the Creative Commons Attribution-NonCommercial-NoDerivs 4.0 International License (CC BY-NC-ND 4.0), which permits the non-commercial replication and distribution of the article with the strict proviso that no changes or edits are made and the original work is properly cited (including links to both the formal publication through the relevant DOI and the license). See: https://creativecommons.org/licenses/by-nc-nd/4.0/.
References
- Bray F, Laversanne M, Sung H, et al. Global cancer statistics 2022: GLOBOCAN estimates of incidence and mortality worldwide for 36 cancers in 185 countries. CA Cancer J Clin 2024;74:229-63. [Crossref] [PubMed]
- Shi P, Li Z, Zhang Y, et al. Surgery or radiotherapy improves survival in elderly patients with early non-small cell lung cancer: A population-based analysis. J Cancer Res Ther 2024;20:1251-7. [Crossref] [PubMed]
- Mukhopadhyay D, Cocco P, Orrù S, et al. The role of MicroRNAs as early biomarkers of asbestos-related lung cancer: A systematic review and meta-analysis. Pulmonology 2025;31:2416792. [Crossref] [PubMed]
- Lou F, Sima CS, Rusch VW, et al. Differences in patterns of recurrence in early-stage versus locally advanced non-small cell lung cancer. Ann Thorac Surg 2014;98:1755-60; discussion 1760-1. [Crossref] [PubMed]
- Taylor MD, Nagji AS, Bhamidipati CM, et al. Tumor recurrence after complete resection for non-small cell lung cancer. Ann Thorac Surg 2012;93:1813-20; discussion 1820-1. [Crossref] [PubMed]
- Uramoto H, Tanaka F. Recurrence after surgery in patients with NSCLC. Transl Lung Cancer Res 2014;3:242-9. [Crossref] [PubMed]
- Heitzer E, Haque IS, Roberts CES, et al. Current and future perspectives of liquid biopsies in genomics-driven oncology. Nat Rev Genet 2019;20:71-88. [Crossref] [PubMed]
- Vandekerckhove O, Cuppens K, Pat K, et al. Liquid Biopsy in Early-Stage Lung Cancer: Current and Future Clinical Applications. Cancers (Basel) 2023;15:2702. [Crossref] [PubMed]
- Siniscalco D, Galderisi U, Peluso G, et al. Circulating microRNAs in Cancer: A 5-Year Update with a Focus on Breast and Lung Cancers. Int J Mol Sci 2024;25:3140. [Crossref] [PubMed]
- Chechlinska M, Kowalewska M, Nowak R. Systemic inflammation as a confounding factor in cancer biomarker discovery and validation. Nat Rev Cancer 2010;10:2-3. [Crossref] [PubMed]
- Halin Bergström S, Lundholm M, Nordstrand A, et al. Rat prostate tumors induce DNA synthesis in remote organs. Sci Rep 2022;12:7908. [Crossref] [PubMed]
- Kowalewska M, Nowak R, Chechlinska M. Implications of cancer-associated systemic inflammation for biomarker studies. Biochim Biophys Acta 2010;1806:163-71. [Crossref] [PubMed]
- McAllister SS, Weinberg RA. The tumour-induced systemic environment as a critical regulator of cancer progression and metastasis. Nat Cell Biol 2014;16:717-27. [Crossref] [PubMed]
- Sanegre S, Lucantoni F, Burgos-Panadero R, et al. Integrating the Tumor Microenvironment into Cancer Therapy. Cancers (Basel) 2020;12:1677. [Crossref] [PubMed]
- Leidinger P, Galata V, Backes C, et al. Longitudinal study on circulating miRNAs in patients after lung cancer resection. Oncotarget 2015;6:16674-85. [Crossref] [PubMed]
- Le HB, Zhu WY, Chen DD, et al. Evaluation of dynamic change of serum miR-21 and miR-24 in pre- and post-operative lung carcinoma patients. Med Oncol 2012;29:3190-7. [Crossref] [PubMed]
- Aushev VN, Zborovskaya IB, Laktionov KK, et al. Comparisons of microRNA patterns in plasma before and after tumor removal reveal new biomarkers of lung squamous cell carcinoma. PLoS One 2013;8:e78649. [Crossref] [PubMed]
- Sromek M, Glogowski M, Chechlinska M, et al. Changes in plasma miR-9, miR-16, miR-205 and miR-486 levels after non-small cell lung cancer resection. Cell Oncol (Dordr) 2017;40:529-36. [Crossref] [PubMed]
- Nicholson AG, Tsao MS, Beasley MB, et al. The 2021 WHO Classification of Lung Tumors: Impact of Advances Since 2015. J Thorac Oncol 2022;17:362-87. [Crossref] [PubMed]
- Gerds TA, Cai T, Schumacher M. The performance of risk prediction models. Biom J 2008;50:457-79. [Crossref] [PubMed]
- Ghajar CM, Bissell MJ. Metastasis: Pathways of parallel progression. Nature 2016;540:528-9. [Crossref] [PubMed]
- Wankhede D, Grover S, Hofman P. Circulating Tumor Cells as a Predictive Biomarker in Resectable Lung Cancer: A Systematic Review and Meta-Analysis. Cancers (Basel) 2022;14:6112. [Crossref] [PubMed]
- Fina E, Federico D, Novellis P, et al. Subpopulations of Circulating Cells with Morphological Features of Malignancy Are Preoperatively Detected and Have Differential Prognostic Significance in Non-Small Cell Lung Cancer. Cancers (Basel) 2021;13:4488. [Crossref] [PubMed]
- Klein CA. Cancer progression and the invisible phase of metastatic colonization. Nat Rev Cancer 2020;20:681-94. [Crossref] [PubMed]
- Rahrmann EP, Shorthouse D, Jassim A, et al. The NALCN channel regulates metastasis and nonmalignant cell dissemination. Nat Genet 2022;54:1827-38. [Crossref] [PubMed]
- Ilie M, Hofman V, Long-Mira E, et al. "Sentinel" circulating tumor cells allow early diagnosis of lung cancer in patients with chronic obstructive pulmonary disease. PLoS One 2014;9:e111597. [Crossref] [PubMed]
- Nordgård O, Singh G, Solberg S, et al. Novel molecular tumor cell markers in regional lymph nodes and blood samples from patients undergoing surgery for non-small cell lung cancer. PLoS One 2013;8:e62153. [Crossref] [PubMed]
- Antunes-Ferreira M, D'Ambrosi S, Arkani M, et al. Tumor-educated platelet blood tests for Non-Small Cell Lung Cancer detection and management. Sci Rep 2023;13:9359. [Crossref] [PubMed]
- Keller A, Leidinger P, Borries A, et al. miRNAs in lung cancer - studying complex fingerprints in patient's blood cells by microarray experiments. BMC Cancer 2009;9:353. [Crossref] [PubMed]
- Zeng XL, Zhang SY, Zheng JF, et al. Altered miR-143 and miR-150 expressions in peripheral blood mononuclear cells for diagnosis of non-small cell lung cancer. Chin Med J (Engl) 2013;126:4510-6.
- Egeblad M, Nakasone ES, Werb Z. Tumors as organs: complex tissues that interface with the entire organism. Dev Cell 2010;18:884-901. [Crossref] [PubMed]
- Heneghan HM, Miller N, Lowery AJ, et al. Circulating microRNAs as novel minimally invasive biomarkers for breast cancer. Ann Surg 2010;251:499-505. [Crossref] [PubMed]
- Kim SY, Jeon TY, Choi CI, et al. Validation of circulating miRNA biomarkers for predicting lymph node metastasis in gastric cancer. J Mol Diagn 2013;15:661-9. [Crossref] [PubMed]
- Yamamoto Y, Kosaka N, Tanaka M, et al. MicroRNA-500 as a potential diagnostic marker for hepatocellular carcinoma. Biomarkers 2009;14:529-38. [Crossref] [PubMed]
- Zhang JX, Yang W, Wu JZ, et al. MicroRNA-32-5p inhibits epithelial-mesenchymal transition and metastasis in lung adenocarcinoma by targeting SMAD family 3. J Cancer 2021;12:2258-67. [Crossref] [PubMed]
- Fink-Neuboeck N, Lindenmann J, Porubsky C, et al. Hazards of Recurrence, Second Primary, or Other Tumor at Ten Years After Surgery for Non-Small-Cell Lung Cancer. Clin Lung Cancer 2020;21:333-40. [Crossref] [PubMed]
- Rice D, Kim HW, Sabichi A, et al. The risk of second primary tumors after resection of stage I nonsmall cell lung cancer. Ann Thorac Surg 2003;76:1001-7; discussion 1007-8. [Crossref] [PubMed]
- Karabegović I, Maas SCE, Shuai Y, et al. Smoking-related dysregulation of plasma circulating microRNAs: the Rotterdam study. Hum Genomics 2023;17:61. [Crossref] [PubMed]
- Takahashi K, Yokota S, Tatsumi N, et al. Cigarette smoking substantially alters plasma microRNA profiles in healthy subjects. Toxicol Appl Pharmacol 2013;272:154-60. [Crossref] [PubMed]
- Schembri F, Sridhar S, Perdomo C, et al. MicroRNAs as modulators of smoking-induced gene expression changes in human airway epithelium. Proc Natl Acad Sci U S A 2009;106:2319-24. [Crossref] [PubMed]
- Steiling K, Ryan J, Brody JS, et al. The field of tissue injury in the lung and airway. Cancer Prev Res (Phila) 2008;1:396-403. [Crossref] [PubMed]
- Soto AM, Sonnenschein C. The cancer puzzle: Welcome to organicism. Prog Biophys Mol Biol 2021;165:114-9. [Crossref] [PubMed]
- Dolgalev I, Zhou H, Murrell N, et al. Inflammation in the tumor-adjacent lung as a predictor of clinical outcome in lung adenocarcinoma. Nat Commun 2023;14:6764. [Crossref] [PubMed]
- Matsutani N, Sawabata N, Yamaguchi M, et al. Does lung cancer surgery cause circulating tumor cells?-A multicenter, prospective study. J Thorac Dis 2017;9:2419-26. [Crossref] [PubMed]
- Demicheli R, Retsky MW, Hrushesky WJ, et al. The effects of surgery on tumor growth: a century of investigations. Ann Oncol 2008;19:1821-8. [Crossref] [PubMed]
- Nallamshetty S, Chan SY, Loscalzo J. Hypoxia: a master regulator of microRNA biogenesis and activity. Free Radic Biol Med 2013;64:20-30. [Crossref] [PubMed]
- Coronel-Hernández J, Delgado-Waldo I, Cantú de León D, et al. HypoxaMIRs: Key Regulators of Hallmarks of Colorectal Cancer. Cells 2022;11:1895. [Crossref] [PubMed]
- Dejima H, Nakanishi H, Kuroda H, et al. Detection of abundant megakaryocytes in pulmonary artery blood in lung cancer patients using a microfluidic platform. Lung Cancer 2018;125:128-35. [Crossref] [PubMed]
- Lefrançais E, Ortiz-Muñoz G, Caudrillier A, et al. The lung is a site of platelet biogenesis and a reservoir for haematopoietic progenitors. Nature 2017;544:105-9. [Crossref] [PubMed]
- Berridge MV, Dong L, Neuzil J. Mitochondrial DNA in Tumor Initiation, Progression, and Metastasis: Role of Horizontal mtDNA Transfer. Cancer Res 2015;75:3203-8. [Crossref] [PubMed]
- Kroemer G, McQuade JL, Merad M, et al. Bodywide ecological interventions on cancer. Nat Med 2023;29:59-74. [Crossref] [PubMed]