Molecular heterogeneity of small cell lung cancer and new therapeutic possibilities: a narrative review of the literature
Introduction
Small cell lung cancer (SCLC) is an extremely aggressive neuroendocrine (NE) disease, accounting for approximately 13% of all lung cancers, with very few therapeutic options, and is closely linked to heavy tobacco use (1,2).
SCLC is characterized by rapid growth and early development of metastases. Patients commonly present respiratory symptoms, including cough, dyspnea, and hemoptysis, with radiological imaging often showing a central pulmonary nodule with extensive mediastinal lymphadenopathy. The majority of SCLC patients present with distant metastases at initial diagnosis, particularly affecting controlateral lung, brain, liver, adrenal glands and bones (3). Histologically, SCLC is composed by diffusely distributed small or intermediate-size cells, with granular chromatin and scant cytoplasm, characterized by high mitotic activity, apoptotic features and extensive necrosis (4). According to the 5th edition of the World Health Organization (WHO) Thoracic Malignancies classification (5), most SCLCs express at least one positive NE marker (chromogranin A, synaptophysin, CD56, and INSM1) on immunohistochemistry (IHC) (6). Genetically, biallelic loss of function of TP53 and RB genes are the two most common alterations detected in SCLCs (7).
Patients with limited stage (LS) disease are treated with concurrent radiotherapy and platinum-based chemotherapy (8). Until a few years ago, the standard first-line treatment for patients with extensive SCLC (ES-SCLC) was chemotherapy with platinum and etoposide, with a progression-free survival (PFS) of 4.3–5.7 months and a median overall survival (OS) of 7.5–10.9 months (9,10).
In ES-SCLC setting, the addition of anti-programmed death-ligand 1 (PD-L1) monoclonal antibodies (atezolizumab or durvalumab) to standard chemotherapy, followed by immunotherapy as maintenance, led to improved clinical outcomes in terms of PFS and OS (11,12), with a long-lasting benefit in a subgroup of patients and an approximate doubling of 2- or 3-year survival rates (13,14). Subsequently, other immune checkpoint inhibitors (ICIs), such as toripalimab, tislelizumab, adebrelimab, and serplulimab confirmed the durable response to combination therapy (15). Recently, also in LS-SCLC the addition of durvalumab after concurrent chemoradiotherapy led to significantly longer PFS and OS, when compared to placebo (16). Nowadays, validated biomarkers of sensitivity or resistance to ICIs are missing. PD-L1 tumor proportion score (TPS), a predictive biomarker for ICIs mostly in non-small cell lung cancer (NSCLC), failed to predict response to chemo-immunotherapy in SCLC (17).
The lack of significant progress in therapeutic options might be attributed to our poor understanding of the biology of this entity. In contrast to the growing trend of personalized treatment of NSCLC patients, SCLC is still approached in clinical practice as a unique disease. The biological heterogeneity of SCLC has started to emerge only during the last few years. Recent advances have revealed a biological and clinical heterogeneity in SCLC, and different subtypes, with potentially different sensitivity to chemotherapy and ICIs, have been identified. Moreover, the rapid development of resistance to both chemotherapy and immunotherapy represents a clinical urgent challenge and the intra-tumoral heterogeneity at baseline and the evolutionary trajectory of the disease during treatment might be a contributing factor (18).
The aim of this review is to provide an overview of genomic alterations involved in SCLC heterogeneity as well as the complexity of immune cell infiltrate that characterizes the tumor microenvironment (TME). We describe the molecular classification into four SCLC subtypes and summarize its potential clinical and therapeutic implications. We present this article in accordance with the Narrative Review reporting checklist (available at https://tlcr.amegroups.com/article/view/10.21037/tlcr-24-755/rc).
Methods
This is a narrative review that reports on previously published articles identified through a search on PubMed. The search terms included “SCLC”, “molecular heterogeneity”, “molecular subtypes”, “SCLC subclassification”, and “biomarkers”. Articles written in English and published between 1981 and October 2024 were thoroughly reviewed. Relevant articles were selected to provide detailed information about the molecular classification of SCLC and its clinical and therapeutic implications. All the articles included are reported in the reference list. The search strategy is detailed and shown in Table 1.
Table 1
Items | Specification |
---|---|
Date of search | September 2023 to October 2024 |
Databases searched | PubMed |
Search terms used | “SCLC”, “molecular heterogeneity”, “molecular subtypes”, “SCLC subclassification”, and “biomarkers” |
Timeframe | From 1981 to October 2024 |
Inclusion and exclusion criteria | Clinical trial, literature review, review paper. Full-text English published articles were included |
Selection process | V.C., S.D., I.T. conducted the selection independently, and consensus was obtained with the authors to include relevant information |
SCLC, small cell lung cancer.
Genomic alterations involved in SCLC carcinogenesis
Genomic analyses of SCLC tumor samples revealed a high incidence of loss-of-function gene alterations (deletions, truncations, and missense mutations), mainly in two suppressor genes, RB and TP53. These alterations were detected in at least 90% to 100% of cases and play a key role in regulating gene transcription, leading to cellular transformation and malignant progression (7,19).
RB inactivation, due to loss-of-function alterations, induces a loss of its ability to inhibit E2F factors with an improvement of uncontrolled cell plasticity and proliferation. On the other hand, p53 is a transcriptional factor involved in the maintenance of genomic stability, either arresting cell cycle or inducing cell apoptosis when an impaired replication has been revealed. Therefore, when it is inactivated, cells accumulate potentially oncogenic mutations.
Mutations in both genes are a prerequisite for SCLC pathogenesis, as confirmed in mouse model lung epithelial cells (20). However, the inactivation of RB and p53 is not enough to drive malignant progression, and other genomic events are needed (21).
Subclassification into four SCLC subtypes
Historical classification in classic/variant and NE high/NE low subtypes
Historically, SCLC was categorized into two primary subtypes: classic and variant subtypes, which were different in both morphological and molecular features (22,23). Classic SCLC cell lines are characterized by an in-vitro growth in non-adherent aggregates, while the variant ones usually grow as loosely aggregated or single cells. Variant SCLC showed a higher aggressivity and an increased resistance to radiation exposure. Occurrence of MYC amplification was more frequent in the variant subtype, often associated with loss of NE markers, including achaete-scute homolog 1 (ASCL1) and neurogenic differentiation 1 (NEUROD1) transcription factors.
In 2018, Zhang et al. (24) developed a 50-gene expression-based score to classify SCLC in NE high and NE low subtypes, partially corresponding to the previously described two morphologies. This score, based on 50 genes highly or negatively correlated with NE differentiation, led to a more detailed characterization of two entities: the NE high phenotype was associated with ASCL1 and/or NEUROD1 expression, while the NE low phenotype showed MYC and Notch activation and a molecular reprogramming toward epithelial to mesenchymal transition (EMT).
At the same time, Huang et al. (25) identified the transcription factor POU class 2 homeobox 3 (POU2F3) as an exclusive regulator of a small SCLC subgroup. This was recognized as an independent third entity, correlated with lack of NE markers and a high chemosensitivity. Conversely, yes-associated protein 1 (YAP1) expression and Hippo signaling activation were identified in a fourth subgroup, with poor NE differentiation, and hypothesized to predict chemo-refractory and decreased patient survival (26).
Classification based on four main transcription regulators
In 2019, Rudin et al. proposed a consensus nomenclature for SCLC molecular subtypes, by collecting data from human tumors, cell lines and mouse models of SCLC. They provided a systematic molecular classification to divide SCLC into four major subtypes (SCLC-A, SCLC-N, SCLC-P, and SCLC-Y), with the last letter meaning the transcription regulator factor most strongly associated with each subtype: ASCL1, NEUROD1, POU2F3, and YAP1, respectively (9-12,27). These subgroups showed differences in morphology, gene expression, immune infiltration, invasiveness, and treatment sensitivity (28,29).
The immunohistochemical and histopathologic classification into four subtypes was confirmed by Baine et al. in a large cohort of patients (30). SCLC-A and SCLC-N represent NE subtypes, with IHC expression of synaptophysin, CD56, chromogranin A, and INSM1. Research findings indicate that a significant proportion of SCLCs (up to 37%) displays co-expression of ASCL1 and NEUROD1, suggesting a potential functional interaction between these transcription factors (30,31). POU2F3 was mutually exclusive of ASCL1 and NEUROD1, while YAP1 was expressed at low levels and was not exclusive of other subtypes.
In 2022, Qu et al. validated the proposed molecular classification of SCLC tumors using reproducible IHC staining and identified interesting associations between subtypes and therapeutic markers (32). They used three human SCLC tumor microarrays (TMAs) containing 194 cores from 146 SCLC tumors. ASCL1, NEUROD1, POU2F3, and YAP1 were the dominant transcription factors in 78.2%, 5.6%, 7%, and 2.8% of the tumors, respectively; on IHC analysis, 6.3% of SCLC tumors were negative for all four markers (NAPYneg). They observed substantial intratumoral heterogeneity, referring to the presence of spatially distinct cell populations into the same tumor: 17.6% of the tumors appeared positive for two subtype markers and 2.8% were positive for three subtype markers. Interestingly, differently from other works (30,33), this study was able to identify a 2.8% YAP-positive SCLC subgroup, probably associated to the LS of the cohort (34). Authors’ results supported the feasibility of using IHC to distinguish SCLC molecular subtypes and suggested that NAPYneg tumors may represent a new molecular subtype.
The IHC classification of SCLC correlates with different transcriptomic patterns. As described by Chan et al. (35), single-cell RNA sequencing offers a unique opportunity to dissect the intratumoral heterogeneity of SCLC and its TME. Starting from IHC subtypes, they described distinct transcriptomic subtypes by using single-cell RNA sequencing. This methodology unraveled a biological complexity that cannot be described by IHC subtypes and supports IHC-based classification of SCLC.
Transcriptional patterns have to be seen as a dynamic and plastic entity: in mouse and human models, tumor progression may be accompanied by a transition from SCLC-A to SCLC-N and SCLC-Y subtypes, along with higher YAP1 expression (36). The ability of NE cells to transition to non-NE cells has been demonstrated by Ireland et al. (36), who speculates a dynamic transcriptional status of SCLC cells based on MYC-NOTCH molecular axis. Indeed, MYC might directly regulate the expression of several NOTCH pathway genes to drive NE cell dedifferentiation, confirming the “plastic” ability of NE-high cells to transition to non-NE cells (37). The four SCLC subtypes and their differences in NE markers and transcriptional patterns are summarized in Figure 1.
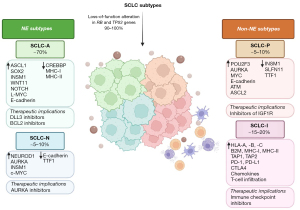
Specific molecular features of SCLC subtypes
The SCLC-A subtype is characterized by high expression of NE markers and involves 70% of human SCLC (38,39). Studies of next-generation sequencing have demonstrated that SOX2 can act both as a human oncogene and a driver of different transcriptional programs in SCLC subgroups (40), and specifically in SCLC-A subgroup, it regulates in an ASCL1-depend manner INSM1 transcription factor, essential for NE differentiation of lung cancer, and WNT11, involved in many mechanisms, including cell fate specification, proliferation, polarity and migration (41,42).
Similarly to SCLC-A, SCLC-N subtype exhibits high expression of NE genes, but it reflects distinct MYC gene expression patterns. The MYC family of transcription factors is frequently amplified in SCLC cell lines and patient tumors (21). The amplification of each MYC family member is exclusive and promotes tumor progression. As supposed by Patel et al. (43) through a RNA-sequencing analysis, SCLC-N detains higher expression of MYC genes, while L-Myc expression was enriched in the SCLC-A subtype. L-Myc and c-Myc expression are responsible for the maintenance of different transcriptional programs that are essential in SCLC subtype determination. Both subgroups appear to share an immunological “cold” profile with low expression of HLA gene and low T-cell infiltration.
POU2F3 is expressed in the cytoplasm of tumor cells in the SCLC-P subtype, predominantly associated with smoking habit, and comports the worse prognosis with low possibility to respond to ICIs alone (34). Both SCLC-P and the “triple negative” SCLC group constitute low-NE subtypes, with negative or minimal labeling for thyroid transcription factor-1 (TTF-1) and NE markers (44).
YAP1 expression is thought to be strictly linked to the reprogramming of SCLC from an NE-high to NE-low phenotype. This process is driven by MYC activation of the Notch signaling pathway. Initially, SCLC-Y was defined as a fourth distinct subtype, but more recent evidence showed that, differently from POU2F3, YAP1 expression was distributed at low levels in all subtypes. Then, at a later time, the validation of SCLC-Y subtype failed, based on IHC and single-cell sequencing (30,31). However, YAP1 expression is negatively correlated with NE marker expression, but positively associated with combined SCLC histology. This led to the hypothesis that YAP1 expression may reveal plasticity within SCLCs switching from an NE to a low-NE state and the development of chemoresistance (36).
Recently, an interesting explanation about the controversial SCLC-Y subtype has been provided by Ng et al. (45). They noted that this subtype showed pathological features similar to SMARCA4-undifferentiated tumors (SMARCA4-UT), including RB1 expression and SMARCA4 and SMARCA2 deficiency. SMARCA4-UT represents a rare mimic of SCLC (46) and exhibits a diffuse and strong expression of YAP1: it is a highly aggressive lung malignancy with lack of epithelial markers. This is consistent with studies that failed to identify an SCLC-Y subtype (31) and support the hypothesis that SCLC-Y is a different and distinct entity from SCLC (32).
SCLC-I subtype
An inflamed subtype of SCLC lacking NEUROD1, ASCL1, and POU2F3 expression (SCLC-I) was recently proposed by Gay et al. to replace the YAP1 subtype (31). High expression of CD8A and CD8B characterizes SCLC-I and suggests greater cytotoxic cell infiltration, including T-cells, macrophages, and natural killer (NK) cells. SCLC-I tumors have the highest immune infiltrate and cytolytic activity, with overexpression of genes involved in inflammatory pathways, like antigen processing and presenting machinery (APM) genes (HLA-A, HLA-B, HLA-C, B2M, TAP1 and TAP2). Moreover, compared to other subtypes, SCLC-I tumors were characterized by a higher expression of immune checkpoint molecules, such as PD-1, PD-L1 and CTLA4, and T-cell attractant chemokines, such as CCL5 and CXCL10. SCLC-I subtype has been shown to be involved in SCLC plasticity and chemoresistance: bioinformatic approaches and data from mouse SCLC cell lines suggest a potential developmental hierarchy among subtypes, with SCLC-A evolving to SCLC-N and then to SCLC-I (36,47), possibly related to treatment and/or progression of disease (48).
Genomic alterations involved in SCLC heterogeneity
Transcription deregulation through different factors alterations has been found to be responsible for SCLC heterogeneity. For example, amplification and overexpression of the MYC gene family, including Myc, Mycl, and Mycn, have been found in 6–25% of primary SCLC tumors. This is correlated with poor outcome, tumor progression, and treatment resistance (48). Studies on human cell lines and the GEMM model of SCLC have demonstrated that Mycl is an SCLC driver (49,50), and its overexpression can affect the NE tumor phenotype (49).
As mentioned above, both mice models and human studies have demonstrated that MYC gene expression is linked to different expression of ASCL1 and NEUROD1, two neurogenic transcription factors (49,50). Furthermore, overexpression is involved in the metastatic process, through direct regulation of Nfib, a member of the nuclear factor I (NFI) family of transcription factors, that in turn is amplified in human SCLC tumors (15%), cell lines (34%) and GEMM mice model (21,49). The MYC amplification plays a critical role also in drug resistance: within relapsed SCLC models, cells that detain more MYC copies received less DNA damage from chemotherapy (51). MYC amplification certainly may explain the evolution of drug resistance, but the translation of this molecular mechanism in patients’ clinical course remains to be clarified.
NOTCH pathway is generally involved in cell-fate decision in many tissues and in GEMM mice model has been shown that its activation was related to strong decrement of tumor and extended survival, suggesting a tumor suppressor’s role (7). NOTCH is closely related to NE differentiation through suppression of ASCL1 expression (21) and promotion of non-NE SCLC fate (52) due to transit amplification and deprogramming of NE stem cells, following lung damage (53). Alteration of NOTCH usually regards 25% of SCLC patients (7).
Similarly, PEA3, a member of the E26 transformation-specific (ETS) transcription factor family, is also involed in maintaining intra-tumoral heterogeneity; its overexpression, mediated by FGF pathway, has been found to promote the invasive migration ability of NE cells (54).
In a large cohort of SCLC samples, also PI3K and RAS/MAPK pathway genes were involved in a small percentage of patients in tumor carcinogenesis. The molecular heterogeneity also concerns a different mutation pattern in different metastatic sites: brain lesions seem to be enriched by PTEN and mTOR alterations, suggesting that the PI3K/AKT/MTOR pathway may play an important role in SCLC brain metastasis (55).
SCLC temporal evolution under treatment pressure
The SCLC heterogeneity also might reflect a temporal tumor evolution under treatment administration. By analyzing 160 tumor samples from 65 patients, George et al. (18) demonstrated that in chemotherapy-sensitive tumors, the rapidly growing and predominant clonal population of cancer cells is eliminated, but when the disease relapses, there is a significant increase in subclonal mutations. Instead, in primarily chemo-refractory tumors, the number of subclonal mutations does not significantly change under treatment. From tumor specimens of patients treated with ICI after chemotherapy, it has been evidenced that earlier ancestral clones that initially disappeared can subsequently re-expand at the time of clinical progression. Gene-damaging alterations in TP53 seem to cover a critical role in this context: the occurrence of co-mutations in TP53 gene and TP73, CREBBP/EP300, or FMN2 genes make tumors poorly responsive to chemotherapy. In the same work, the authors do not find out a correlation between MYC family genes and drug resistance (18).
The SCLC heterogeneity under treatment pressure has also been confirmed by analyzing DNA methylation on both tumor tissue and circulating tumor DNA (ctDNA) in a cohort of 179 SCLC patients (56). Besides transcriptional classification, a DNA methylation-based method can be used as a surrogate of gene expression to develop a prognostic signature and to provide information about tumor evolution. In a large subset of patients, the promoter methylation levels changed between baseline and plasma samples collected at disease progression, translating into a switch from SCLC-A to SCLC-I subtypes. Differences in the promoter methylation of immune-related genes, such as CXCL12, CIITA, STAT1, and IFN-α and IFN-γ receptors, highlighted a shift toward an immune phenotype.
TME immune profile
TME is constituted by a complex network of cell types within the tumor tissue, involving immune and stromal cells. The interactions within different (immune, stromal and tumor) cell populations are mediated by cytokines and metabolites and influence the tumor response to chemotherapy and immunotherapy.
The development of SCLC is characterized by a large amount of potentially immunogenic neoantigens, due to the close correlation of this disease with smoking habit (57). Despite the elevated immunogenicity and the high tumor mutational burden (TMB), SCLC is historically considered an immune-cold disease (58,59).
The proportion of monocytes, activated dendritic cells (DCs), and neutrophils in SCLC tissue is lower than in normal lung tissue (60). Moreover, antigen presentation mechanisms are defective in SCLC, because of an absent or lower expression of major histocompatibility complex (MHC) I or MHC II (61,62).
Tumor cells within SCLC tissues are responsible for the production and secretion of several chemokines and cytokines, such as CD156 and IL-15. These molecules exert an inhibitory effect on tumor-infiltrating lymphocytes (TILs), promoting T cells differentiation to regulatory T (Treg) cells (63), while CD4+ TILs are downregulated in SCLC, resulting in an immune-suppressive microenvironment. Regarding cytotoxic activity, several studies showed a high CD8+ TIL density within SCLC tumor tissues (64) and a high rate of CD8+ TILs is significantly associated with a favorable PFS and OS (38,65,66), also in patients treated with ICIs (64). Recently, the CXCL9-CXL10 chemokine axis has been suggested as a potential biomarker for predicting ICI efficacy. Indeed, co-expressing CCL5 in tumor cells and CXCL9/CXCL10 in immune cells enhanced TIL infiltration: high CCL5 expression indicates better survival in SCLC patients treated with ICIs, so CCL5 might represent a promising predictive biomarker (67).
The role of PD-L1 and TMB
PD-L1 expression is reported to be lower in SCLC cells compared to NSCLC (68). Recent studies assessing the combination of chemotherapy with a PD-1/PD-L1 inhibitor failed to show different outcomes according to PD-L1 expression (69-71). Interestingly, in an exploratory analysis of the CASPIAN trial, a greater OS benefit was observed only in the subgroup of patients treated with a combination of durvalumab and tremelimumab and with PD-L1 expression ≥1% with respect to tumors with PD-L1 <1% (71). Despite this, to date, PD-L1 expression in the SCLC setting is not considered a reliable predictive biomarker, as a similar survival benefit of chemo-immunotherapy over placebo plus chemotherapy was observed independently by PD-L1 subgroups in CASPIAN and Impower133 trials (69-71).
From exploratory analyses on blood samples in the Impower133 trial and on tissue samples in the CASPIAN trial, a high TMB does not appear to be predictive of ICIs response (11,72). Indeed, Paz-Ares et al. (71) reported a similar OS benefit for patients treated within CASPIAN trial regardless tissue TMB status. Similarly, in an exploratory analysis from SCLC patients enrolled in Impower133 trial, a high or low blood-based TMB value was not correlated with PFS or OS rate, showing a consistent benefit in all subgroups (11).
Distinction in immune “hot” and “cold” tumors
Easy-to-use clinical predictive factors for chemo-immunotherapy are still lacking, so this reflects the molecular complexity of different SCLC subgroups. Besides adaptive immune system, also innate immune cells are involved within TME. NK cells, responsible for cytotoxic activity against tumor cells, were found to be reduced in SCLC, while M2-macrophages, correlated with a local immunosuppressive effect, represent one of the most abundant cell types in SCLC (73), thus contributing to immune escape (74). An abundance of M2-macrophages within SCLC tumor tissues is a validated predictor of a shorter OS (65,75).
The heterogeneity of SCLC, in addition to tumor cell components, is also reflected in the TME compartment; as a matter of fact, several articles in the literature attempted to subclassify this entity according to the immunophenotypic profile. In a retrospective study involving 63 patients with ES-SCLC treated with a combination of chemotherapy and ICIs, Kanemura et al. (76) identified a subgroup of “inflamed-patients” (based on PD-L1 expression and CD8+ TIL density) receiving a greater benefit in PFS, compared to “non-inflamed patients”. Through an immune-related gene expression profiling (irGEP), they showed that “inflamed tumors” are characterized by increased stimulation of molecular pathways related to costimulatory signaling (including CD48, CD80, CD274, IL18, IL2RA, and IL15), cytokine and chemokine signaling (including CXCL10, IL10RA, and JAK3) and antigen presentation.
Also patients with the “immune-enriched” (IE) SCLC subtype, as defined by Yang et al. (77), showed a more favorable outcome, in terms of disease-free survival (DFS) and OS. By evaluating resected tumor samples from 59 LS-SCLC patients and by integrating transcriptomics and protein expression analysis, they distinguished two sub-groups: an IE-subtype and an immune-deprived (ID)-subtype, the last one characterized by lack of immune infiltration and a proliferative phenotype. In this cohort of resected patients treated with adjuvant therapy (chemo or radiotherapy), authors developed a computational index for the CCL5/CXCL9 chemokine axis, that showed a promising application in differentiating IE-tumors from ID-tumors.
Referring to genomic and immunohistochemical classification in SCLC-A, SCLC-N, SCLC-P, and SCLC-Y (27), several evidences showed that non-NE tumors can be considered an “immune-hot” subtype according to immune cell populations. An “immune-cold” microenvironment is generally characteristic of NE tumors (including SCLC-A and SCLC-N subtypes) (78) and is correlated to a depletion of cytotoxic T cells and enrichment in immune-suppressive Treg cells (35). On the other side, non-NE SCLC tumors are characterized by higher expression of PD-L1 and immune-related genes expression, associated with antigen presentation (B2M, HLA genes), IFN-γ signaling, cytotoxic T-cell activity, and adaptive immune resistance (32,79,80). Patients with non-NE tumors receiving ICIs derived greater clinical benefit compared to those with NE tumors (28), suggesting that the immune environment of SCLC has an impact on efficacy and response to ICIs (78).
Rudin et al. (29) conducted an exploratory post hoc analysis of CheckMate 032 trial on tumor samples of SCLC patients treated with nivolumab monotherapy or nivolumab combined with ipilimumab, after progression to platinum-based chemotherapy (81,82). In both cohorts of patients, CD8+ tissue infiltration and a higher MHC-I expression were significantly associated with improved survival. Moreover, an antigen presentation signature correlated with CD8+ positivity was found to be enriched in SCLC-Y subtype. Tumors from patients with durable benefit from ICIs presented molecular patterns associated with inflammation gene expression, NK cell activation, and IFN-γ signaling.
As mentioned above, Gay et al. (31) identified a SCLC subtype called “SCLC-I”, to replace the YAP1 subtype. Although the IMpower 133 trial was not statistically powered for subtype-specific analyses, a trend toward improved OS from chemotherapy and atezolizumab was noted in SCLC-I compared to other subtypes, which was not observed in the chemotherapy arm (31). The higher benefit from immunotherapy observed in SCLC-I is related to antigen processing MHC-I and APM expression (83), as evidenced by clinical and preclinical studies (61,84).
Recently, Wei et al. (85) identified a subgroup of SCLC called “Immunity_H”, characterized by a large population of immune cells and a high expression of PD-1, PD-L1, and CTLA4. Also, a high infiltration of CD8+ T cells, NK cells, dendritic cells, and macrophages was found, meaning a context of anti-tumor activity and pro-inflammatory setting. The “Immunity_H” subtype seems to be closely similar to the SCLC-I subtype, described by Gay et al. and both studies referred to a “hot” SCLC subgroup.
Similarly, Tosi et al. (86) also attempted to characterize an immune cell infiltrate able to predict the highest benefit from chemo-immunotherapy in SCLC patients. By analyzing 42 tumor samples from SCLC patients receiving ICIs, they found that an increased density of B lymphocytes and ASCL1+ tumor cells, as well as a higher expression of exhausted CD8-related genes in TME was related to a longer survival in SCLC patients. By combining this information with the presence of CD163+ macrophages close to tumor cells, it has been developed a combined score to stratify patients’ outcome.
Clinical and therapeutic implications of SCLC molecular classification
The potential role, prognostic and predictive, of NE subtype classification is not well determined. SCLC-A, SCLC-N, SCLC-P, and SCLC-I have been shown to have distinct therapeutic vulnerabilities. SCLC-A and SCLC-N are associated with immune cold tumors, based on low levels of immune cell-related RNA expression. In contrast, low NE subtypes are associated with increased immunogenicity, in other words, ‘hot’ or ‘immune oasis’ phenotype; consequently, low NE SCLC patients may more likely respond to immunotherapies (34,87-89). However, none of the four distinct subtypes detain a clear prognostic role.
Recently, Lissa et al. failed to find significant difference in OS between the SCLC subtypes. However, authors showed that NE tumors preferentially respond to replication stress-targeted therapies, while patients affected by non-NE SCLC and treated with ICIs derived greater benefit when compared to those with NE tumors (28).
A small percentage of SCLC patients experience durable responses to ICIs. Currently, there are no biomarkers able to predict a response to ICIs in SCLC. Identifying which patients will significantly benefit from ICIs, and which will not, remains a critical unmet need.
Using data from this Impower133 trial, Gay et al. retrospectively stratified survival data by SCLC subtype: an OS benefit was showen in the SCLC-I group compared to all others in the chemoimmunotherapy arm [hazard ratio (HR), 0.57; 95% confidence interval (CI): 0.32–0.99], but not in the chemotherapy plus placebo arm (HR, 0.75; 95% CI: 0.46–1.22) (31). From the same clinical trial, Liu et al. demonstrated that the largest proportion of long-term survivors (defined as survivors after 18 months from randomization) receiving chemoimmunotherapy was found in the SCLC-I group (28%, with respect to 11% of non-long-term survivors) (90). Similar data comes from subgroup analyses of the CASPIAN study: the greatest OS benefit from chemoimmunotherapy resulted in SCLC-I patients, with a median OS of 24.0 months, compared to 12.1 and 11.5 months of SCLC-N and SCLC-A subtypes, respectively (HR, 0.55; 95% CI: 0.40–0.78) (91). These findings impressively demonstrate the potential of the SCLC-I subtype as a predictive biomarker for immune checkpoint blockade response in SCLC. Conversely, the SCLC-N subtype detain the most immunosuppressive TME and, therefore, may derive no benefit from ICIs (35). It is important to note that SCLC subtyping is an evolving area, and newer subtypes and the extent of intra-tumoral heterogeneity is only beginning to be understood.
Potential therapeutic perspectives according to SCLC molecular classification
In the last ten years, the introduction of a combination of ICIs with chemotherapy represented the most important and practice-changing novelty in the SCLC setting. Recent ongoing studies are focused on new drugs directed against a specific molecular target or modulating the immune system, according to SCLC subtype classification (Table 2).
Table 2
Trial name | Intervention | Setting | Phase | Design | Planned participant enrollment | Status | Primary objective |
---|---|---|---|---|---|---|---|
NCT03319940 | Tarlatamab +/− pembrolizumab | Progression to platinum-based chemotherapy | I | Non-randomized, open-label | 392 | Recruiting | TEAE, DLT |
NCT05060016, DeLLphi-301 | Tarlatamab | Progression to platinum-based regimen and at least another prior line of therapy | II | Randomized, open-label | 222 | Active, not recruiting | ORR, TEAE, serum concentrations of tarlatamab |
NCT05361395 | Tarlatamab + carboplatin + etoposide + ICI (atezolizumab or durvalumab) | First-line | Ib | Non-randomized, open-label | 340 | Recruiting | DLT, TEAE |
NCT06211036, DeLLphi-305 | Durvalumab +/− tarlatamab | First-line | III | Randomized, open-label | 550 | Recruiting | OS |
NCT05740566, DeLLphi-304 | Tarlatamab vs. standard of care | Second-line | III | Randomized, open-label | 490 | Recruiting | OS |
NCT05623319 | Pembrolizumab + olaparib | Maintenance to first-line | II | Single group, open-label | 60 | Recruiting | PFS |
NCT04728230, PRIO trial | Carboplatin + etoposide + durvalumab + olaparib + RT | First-line | I/II | Single group, open-label | 63 | Recruiting | DLT |
NCT04334941 | Atezolizumab +/− talazoparib | Maintenance to first-line | II | Randomized, open-label | 94 | Active, not recruiting | PFS |
NCT03830918 | Atezolizumab +/− temozolomide and niraparib | Maintenance to first-line | Ib/II | Randomized, open-label | 74 | Recruiting | RP2D, PFS |
SCLC, small cell lung cancer; TEAE, treatment-emergent adverse events; DLT, dose-limiting toxicity; ORR, objective response rate; ICI, immune checkpoint inhibitor; OS, overall survival; PFS, progression-free survival; RT, radiotherapy; RP2D, recommended phase II dose.
The expression of DLL3 protein on the tumor cell surface is linked to the expression of ASCL1, so it may represent an interesting therapeutic target particularly for SCLC-A subtype. Among antibody-drug conjugates, rovalpituzumab is the first DLL3-targeted antibody that, after initial promising results, did not show an effective activity in SCLC (92,93). Subsequently, a bispecific T-cell engager molecule directed to the same molecule, tarlatamab, showed a good antitumoral activity with durable objective response rate (ORR) in phase I and phase II clinical trials involving pretreated SCLC patients (NCT03319940 and NCT05060016, DeLLphi-301) (94,95). Tarlatamab is a highly specific molecule, that binds to DLL3 on tumor cells and to CD3 on T cells and induces a cell-mediated tumor cell lysis. Moreover, DLL3 may represent along with clinical parameters and PD-L1 expression, an interesting independent risk factor for prognosis in SCLC patients, as suggested in a nomogram developed by Zhao et al. (96). A phase I trial with a combination of tarlatamab and chemo-immunotherapy in first line and a phase III trial with tarlatamab as first-line maintenance or second line are now ongoing (NCT05361395, NCT06211036, NCT05740566).
Among drugs specifically directed against molecular pathways altered in SCLC-A subtype, Bcl-2 inhibitors (NCT03387332 and NCT03080311) (97), histone deacetylase inhibitors (NCT03345485) (98), and hedgehog inhibitors (99) showed promising results and a manageable safety profile.
MYC gene amplification cooperates with RB1 and TP53 loss of function to promote SCLC tumor cell aggressiveness. MYC alteration is often associated with SCLC-N subtype (49). As demonstrated in a phase II study (100), a subset of patients with c-Myc expression showed an improved ORR and PFS with alisertib [an aurora kinase A (AURKA) inhibitor] in combination with paclitaxel, respect to paclitaxel alone, suggesting an enhanced sensitivity in SCLC-N subgroup. The phase II PUMA-ALI-4201 trial is now ongoing to investigate potential efficacy of alisertib as second-line after chemo-immunotherapy (NCT06095505).
A potential target for SCLC-P patients may be represented by insulin-like growth factor 1 receptor (IGF-1R), due to its overexpression in this subtype (25). The combination of chemotherapy and dalotuzumab, an IGF-1R inhibitor, showed promising results, achieving an ORR of 67% in a phase I study (101). In this subgroup also polyADP ribose polymerase (PARP) inhibitors seem to be a potential therapeutic option. Several studies evaluated the efficacy of PARP inhibitors, such as veliparib or olaparib, in association with temozolomide, reporting significative response rate (ORR about 40% in pre-treated patients) (102,103). Moreover, veliparib combined with platinum-based chemotherapy as first-line confers a greater benefit in terms of PFS, especially for SLFN11 positive patients (104). Several ongoing studies evaluating combinations of a PARP inhibitor with chemo-immunotherapy or ICIs alone are shown in Table 2.
As described above, SCLC-I subtype represents a minority of this aggressive disease that potentially receives a long-last benefit from chemo-immunotherapy. Nowadays, in clinical practice, combination of platinum-based chemotherapy and ICIs represents the “one-fit-all” strategy, but trial ongoing clinical trials will try to overcome resistance to ICIs by adding combination with target drugs (e.g., PARP inhibitors, anti-DLL3 drugs).
Despite the benefit in a minority of patients, SCLC remains characterized by a dismal prognosis in most cases. In recent preclinical and clinical studies, all efforts aim to distinguish different SCLC subtypes, with the goal of providing patients with targeted treatments and prolonging the benefits of chemotherapy and ICIs. The ultimate goal is to introduce a personalized treatment approach in SCLC setting as well, although to date this is not feasible in clinical practice. Tumoral plasticity and temporal heterogeneity of SCLC cells as well as enrollment of unselected patient group in clinical trial makes targeted treatments difficult to realize. Future studies will focus on deeper understanding of SCLC molecular characterization and on identification of distinct key molecular pathways, responsible for tumor development and intratumoral heterogeneity.
Conclusions
The addition of ICIs to standard chemotherapy significantly improves clinical outcomes only in a minority of SCLC patients. Nowadays, differently from other histology such as NSCLC, no reliable and easy-to-use biomarkers are known to be predictive of disease response. Unlike SCLC, which is now clinically viewed as a single entity, tumor molecular heterogeneity, TME complexity, and tumor dynamic plasticity may significantly impact sensitivity to various therapeutic approaches. Indeed, recent evidence showed that features of SCLC subtypes based on transcriptional classification may reflect on different TME composition and on different response to ICIs. Advances in SCLC molecular characterization and the development of drugs specifically directed against molecular pathways might improve patients’ outcome in the next future and help to personalize treatment also in this complex disease.
Acknowledgments
None.
Footnote
Reporting Checklist: The authors have completed the Narrative Review reporting checklist. Available at https://tlcr.amegroups.com/article/view/10.21037/tlcr-24-755/rc
Peer Review File: Available at https://tlcr.amegroups.com/article/view/10.21037/tlcr-24-755/prf
Funding: None.
Conflicts of Interest: All authors have completed the ICMJE uniform disclosure form (available at https://tlcr.amegroups.com/article/view/10.21037/tlcr-24-755/coif). L.R. received honoraria from Eli-Lilly, Roche, Pfizer, Astra-Zeneca, Boehringer, Bristol-Myers Squibb. R.B. reported serving as a consultant/advisory board member for AstraZeneca, Boehringer Ingelheim, Novartis, Merck, Otsuka, MSD, Eli Lilly, Roche, Amgen, GSK, Eisai and BMS. M.T. received speakers’ and consultants’ fee from Astra-Zeneca, Pfizer, Eli-Lilly, BMS, Novartis, Roche, MSD, Boehringer Ingelheim, Takeda, Amgen, Merck, Sanofi, Janssen, Daiichi Sankyo. M.T. received institutional research grants from Astra-Zeneca, Boehringer Ingelheim and Roche. M.T. received travel support from Amgen and Takeda. The other authors have no conflicts of interest to declare.
Ethical Statement: The authors are accountable for all aspects of the work in ensuring that questions related to the accuracy or integrity of any part of the work are appropriately investigated and resolved.
Open Access Statement: This is an Open Access article distributed in accordance with the Creative Commons Attribution-NonCommercial-NoDerivs 4.0 International License (CC BY-NC-ND 4.0), which permits the non-commercial replication and distribution of the article with the strict proviso that no changes or edits are made and the original work is properly cited (including links to both the formal publication through the relevant DOI and the license). See: https://creativecommons.org/licenses/by-nc-nd/4.0/.
References
- Huang R, Wei Y, Hung RJ, et al. Associated Links Among Smoking, Chronic Obstructive Pulmonary Disease, and Small Cell Lung Cancer: A Pooled Analysis in the International Lung Cancer Consortium. EBioMedicine 2015;2:1677-85. [Crossref] [PubMed]
- Govindan R, Page N, Morgensztern D, et al. Changing epidemiology of small-cell lung cancer in the United States over the last 30 years: analysis of the surveillance, epidemiologic, and end results database. J Clin Oncol 2006;24:4539-44. [Crossref] [PubMed]
- Travis WD. Pathology and diagnosis of neuroendocrine tumors: lung neuroendocrine. Thorac Surg Clin 2014;24:257-66. [Crossref] [PubMed]
- Sung S, Heymann JJ, Politis MG, et al. Small Biopsy and Cytology of Pulmonary Neuroendocrine Neoplasms: Brief Overview of Classification, Immunohistochemistry, Molecular Profiles, and World Health Organization Updates. Adv Anat Pathol 2022;29:329-36. [Crossref] [PubMed]
- WHO Classification of Tumours Editorial Board. Thoracic Tumours. WHO Classification of Tumours, 5th Edition, Volume 5. 2021. Available online: https://publications.iarc.fr/Book-And-Report-Series/Who-Classification-Of-Tumours/Thoracic-Tumours-2021
- Rekhtman N. Lung neuroendocrine neoplasms: recent progress and persistent challenges. Mod Pathol 2022;35:36-50. [Crossref] [PubMed]
- George J, Lim JS, Jang SJ, et al. Comprehensive genomic profiles of small cell lung cancer. Nature 2015;524:47-53. [Crossref] [PubMed]
- Dingemans AC, Früh M, Ardizzoni A, et al. Small-cell lung cancer: ESMO Clinical Practice Guidelines for diagnosis, treatment and follow-up☆. Ann Oncol 2021;32:839-53. [Crossref] [PubMed]
- Farago AF, Keane FK. Current standards for clinical management of small cell lung cancer. Transl Lung Cancer Res 2018;7:69-79. [Crossref] [PubMed]
- Schabath MB, Nguyen A, Wilson P, et al. Temporal trends from 1986 to 2008 in overall survival of small cell lung cancer patients. Lung Cancer 2014;86:14-21. [Crossref] [PubMed]
- Horn L, Mansfield AS, Szczęsna A, et al. First-Line Atezolizumab plus Chemotherapy in Extensive-Stage Small-Cell Lung Cancer. N Engl J Med 2018;379:2220-9. [Crossref] [PubMed]
- Paz-Ares L, Dvorkin M, Chen Y, et al. Durvalumab plus platinum-etoposide versus platinum-etoposide in first-line treatment of extensive-stage small-cell lung cancer (CASPIAN): a randomised, controlled, open-label, phase 3 trial. Lancet 2019;394:1929-39. [Crossref] [PubMed]
- Paz-Ares L, Chen Y, Reinmuth N, et al. Durvalumab, with or without tremelimumab, plus platinum-etoposide in first-line treatment of extensive-stage small-cell lung cancer: 3-year overall survival update from CASPIAN. ESMO Open 2022;7:100408. [Crossref] [PubMed]
- Liu SV, Dziadziuszko R, Sugawara S, et al. OA01. 04 five-year survival in patients with ES-SCLC treated with atezolizumab in IMpower133: imbrella a extension study results. J Thorac Oncol 2023;18:S44-5.
- Yang C, Xuan T, Gong Q, et al. Efficacy and safety of novel immune checkpoint inhibitor-based combinations versus chemotherapy as first-line treatment for patients with extensive-stage small cell lung cancer: A network meta-analysis. Thorac Cancer 2024;15:1246-62. [Crossref] [PubMed]
- Cheng Y, Spigel DR, Cho BC, et al. Durvalumab after Chemoradiotherapy in Limited-Stage Small-Cell Lung Cancer. N Engl J Med 2024;391:1313-27. [Crossref] [PubMed]
- Rolfo C, Russo A. In Search of Lost Biomarker for Immunotherapy in Small Cell Lung Cancer. Clin Cancer Res 2024;30:652-4. [Crossref] [PubMed]
- George J, Maas L, Abedpour N, et al. Evolutionary trajectories of small cell lung cancer under therapy. Nature 2024;627:880-9. [Crossref] [PubMed]
- Sherr CJ, McCormick F. The RB and p53 pathways in cancer. Cancer Cell 2002;2:103-12. [Crossref] [PubMed]
- Meuwissen R, Linn SC, Linnoila RI, et al. Induction of small cell lung cancer by somatic inactivation of both Trp53 and Rb1 in a conditional mouse model. Cancer Cell 2003;4:181-9. [Crossref] [PubMed]
- Kim DW, Kim KC, Kim KB, et al. Transcriptional deregulation underlying the pathogenesis of small cell lung cancer. Transl Lung Cancer Res 2018;7:4-20. [Crossref] [PubMed]
- Carney DN, Gazdar AF, Bepler G, et al. Establishment and identification of small cell lung cancer cell lines having classic and variant features. Cancer Res 1985;45:2913-23.
- Gazdar AF, Carney DN, Minna JD. In vitro study of the biology of small cell carcinoma of the lung. Yale J Biol Med 1981;54:187-93.
- Zhang W, Girard L, Zhang YA, et al. Small cell lung cancer tumors and preclinical models display heterogeneity of neuroendocrine phenotypes. Transl Lung Cancer Res 2018;7:32-49. [Crossref] [PubMed]
- Huang YH, Klingbeil O, He XY, et al. POU2F3 is a master regulator of a tuft cell-like variant of small cell lung cancer. Genes Dev 2018;32:915-28. [Crossref] [PubMed]
- McColl K, Wildey G, Sakre N, et al. Reciprocal expression of INSM1 and YAP1 defines subgroups in small cell lung cancer. Oncotarget 2017;8:73745-56. [Crossref] [PubMed]
- Rudin CM, Poirier JT, Byers LA, et al. Molecular subtypes of small cell lung cancer: a synthesis of human and mouse model data. Nat Rev Cancer 2019;19:289-97. [Crossref] [PubMed]
- Lissa D, Takahashi N, Desai P, et al. Heterogeneity of neuroendocrine transcriptional states in metastatic small cell lung cancers and patient-derived models. Nat Commun 2022;13:2023. [Crossref] [PubMed]
- Rudin CM, Balli D, Lai WV, et al. Clinical Benefit From Immunotherapy in Patients With SCLC Is Associated With Tumor Capacity for Antigen Presentation. J Thorac Oncol 2023;18:1222-32. [Crossref] [PubMed]
- Baine MK, Hsieh MS, Lai WV, et al. SCLC Subtypes Defined by ASCL1, NEUROD1, POU2F3, and YAP1: A Comprehensive Immunohistochemical and Histopathologic Characterization. J Thorac Oncol 2020;15:1823-35. [Crossref] [PubMed]
- Gay CM, Stewart CA, Park EM, et al. Patterns of transcription factor programs and immune pathway activation define four major subtypes of SCLC with distinct therapeutic vulnerabilities. Cancer Cell 2021;39:346-360.e7. [Crossref] [PubMed]
- Qu S, Fetsch P, Thomas A, et al. Molecular Subtypes of Primary SCLC Tumors and Their Associations With Neuroendocrine and Therapeutic Markers. J Thorac Oncol 2022;17:141-53. [Crossref] [PubMed]
- Pearsall SM, Humphrey S, Revill M, et al. The Rare YAP1 Subtype of SCLC Revisited in a Biobank of 39 Circulating Tumor Cell Patient Derived Explant Models: A Brief Report. J Thorac Oncol 2020;15:1836-43. [Crossref] [PubMed]
- Owonikoko TK, Dwivedi B, Chen Z, et al. YAP1 Expression in SCLC Defines a Distinct Subtype With T-cell-Inflamed Phenotype. J Thorac Oncol 2021;16:464-76. [Crossref] [PubMed]
- Chan JM, Quintanal-Villalonga Á, Gao VR, et al. Signatures of plasticity, metastasis, and immunosuppression in an atlas of human small cell lung cancer. Cancer Cell 2021;39:1479-1496.e18. [Crossref] [PubMed]
- Ireland AS, Micinski AM, Kastner DW, et al. MYC Drives Temporal Evolution of Small Cell Lung Cancer Subtypes by Reprogramming Neuroendocrine Fate. Cancer Cell 2020;38:60-78.e12. [Crossref] [PubMed]
- Ng J, Sutherland KD. NOTCH Your Usual Suspect: MYC Charged with Controlling Neuroendocrine Cell-Fate in Small Cell Lung Cancer. Cancer Cell 2020;38:17-20. [Crossref] [PubMed]
- Ding XL, Su YG, Yu L, et al. Clinical characteristics and patient outcomes of molecular subtypes of small cell lung cancer (SCLC). World J Surg Oncol 2022;20:54. [Crossref] [PubMed]
- Ito T, Udaka N, Ikeda M, et al. Significance of proneural basic helix-loop-helix transcription factors in neuroendocrine differentiation of fetal lung epithelial cells and lung carcinoma cells. Histol Histopathol 2001;16:335-43. [Crossref] [PubMed]
- Rudin CM, Durinck S, Stawiski EW, et al. Comprehensive genomic analysis identifies SOX2 as a frequently amplified gene in small-cell lung cancer. Nat Genet 2012;44:1111-6. [Crossref] [PubMed]
- Pongracz JE, Stockley RA. Wnt signalling in lung development and diseases. Respir Res 2006;7:15. [Crossref] [PubMed]
- Fujino K, Motooka Y, Hassan WA, et al. Insulinoma-Associated Protein 1 Is a Crucial Regulator of Neuroendocrine Differentiation in Lung Cancer. Am J Pathol 2015;185:3164-77. [Crossref] [PubMed]
- Patel AS, Yoo S, Kong R, et al. Prototypical oncogene family Myc defines unappreciated distinct lineage states of small cell lung cancer. Sci Adv 2021;7:eabc2578. [Crossref] [PubMed]
- Wang Y, Jin Y, Shen X, et al. POU2F3: A Sensitive and Specific Diagnostic Marker for Neuroendocrine-low/negative Small Cell Lung Cancer. Am J Surg Pathol 2023;47:1059-66. [Crossref] [PubMed]
- Ng J, Cai L, Girard L, et al. Molecular and Pathologic Characterization of YAP1-Expressing Small Cell Lung Cancer Cell Lines Leads to Reclassification as SMARCA4-Deficient Malignancies. Clin Cancer Res 2024;30:1846-58. [Crossref] [PubMed]
- Rekhtman N. All That Is Small Is Not a Small-Cell Carcinoma: Thoracic SMARCA4-Deficient Undifferentiated Tumors Masquerading as SCLC. Clin Cancer Res 2024;30:1708-11. [Crossref] [PubMed]
- Wooten DJ, Groves SM, Tyson DR, et al. Systems-level network modeling of Small Cell Lung Cancer subtypes identifies master regulators and destabilizers. PLoS Comput Biol 2019;15:e1007343. [Crossref] [PubMed]
- Bai R, Li L, Chen X, et al. Advances in novel molecular typing and precise treatment strategies for small cell lung cancer. Chin J Cancer Res 2021;33:522-34. [Crossref] [PubMed]
- Mollaoglu G, Guthrie MR, Böhm S, et al. MYC Drives Progression of Small Cell Lung Cancer to a Variant Neuroendocrine Subtype with Vulnerability to Aurora Kinase Inhibition. Cancer Cell 2017;31:270-85. [Crossref] [PubMed]
- Polley E, Kunkel M, Evans D, et al. Small Cell Lung Cancer Screen of Oncology Drugs, Investigational Agents, and Gene and microRNA Expression. J Natl Cancer Inst 2016;108:djw122. [Crossref] [PubMed]
- Pal Choudhuri S, Girard L, Lim JYS, et al. Acquired Cross-Resistance in Small Cell Lung Cancer due to Extrachromosomal DNA Amplification of MYC Paralogs. Cancer Discov 2024;14:804-27. [Crossref] [PubMed]
- Lim JS, Ibaseta A, Fischer MM, et al. Intratumoural heterogeneity generated by Notch signalling promotes small-cell lung cancer. Nature 2017;545:360-4. [Crossref] [PubMed]
- Ouadah Y, Rojas ER, Riordan DP, et al. Rare Pulmonary Neuroendocrine Cells Are Stem Cells Regulated by Rb, p53, and Notch. Cell 2019;179:403-416.e23. [Crossref] [PubMed]
- Oh S, Shin S, Janknecht R. ETV1, 4 and 5: an oncogenic subfamily of ETS transcription factors. Biochim Biophys Acta 2012;1826:1-12. [Crossref] [PubMed]
- Sivakumar S, Moore JA, Montesion M, et al. Integrative Analysis of a Large Real-World Cohort of Small Cell Lung Cancer Identifies Distinct Genetic Subtypes and Insights into Histologic Transformation. Cancer Discov 2023;13:1572-91. [Crossref] [PubMed]
- Heeke S, Gay CM, Estecio MR, et al. Tumor- and circulating-free DNA methylation identifies clinically relevant small cell lung cancer subtypes. Cancer Cell 2024;42:225-237.e5. [Crossref] [PubMed]
- Pleasance ED, Stephens PJ, O'Meara S, et al. A small-cell lung cancer genome with complex signatures of tobacco exposure. Nature 2010;463:184-90. [Crossref] [PubMed]
- Sabari JK, Lok BH, Laird JH, et al. Unravelling the biology of SCLC: implications for therapy. Nat Rev Clin Oncol 2017;14:549-61. [Crossref] [PubMed]
- Facchinetti F, Marabelle A, Rossi G, et al. Moving Immune Checkpoint Blockade in Thoracic Tumors beyond NSCLC. J Thorac Oncol 2016;11:1819-36. [Crossref] [PubMed]
- Chen MY, Zeng YC, Zhao XH. Chemotherapy- and Immune-Related Gene Panel in Prognosis Prediction and Immune Microenvironment of SCLC. Front Cell Dev Biol 2022;10:893490. [Crossref] [PubMed]
- Mahadevan NR, Knelson EH, Wolff JO, et al. Intrinsic Immunogenicity of Small Cell Lung Carcinoma Revealed by Its Cellular Plasticity. Cancer Discov 2021;11:1952-69. [Crossref] [PubMed]
- He Y, Rozeboom L, Rivard CJ, et al. MHC class II expression in lung cancer. Lung Cancer 2017;112:75-80. [Crossref] [PubMed]
- Wang W, Hodkinson P, McLaren F, et al. Small cell lung cancer tumour cells induce regulatory T lymphocytes, and patient survival correlates negatively with FOXP3+ cells in tumour infiltrate. Int J Cancer 2012;131:E928-37. [Crossref] [PubMed]
- Sun Y, Zhai C, Chen X, et al. Characterization of PD-L1 protein expression and CD8+ tumor-infiltrating lymphocyte density, and their associations with clinical outcome in small-cell lung cancer. Transl Lung Cancer Res 2019;8:748-59. [Crossref] [PubMed]
- Li F, Yang Y, Xu Y, et al. Comparative study of the genomic landscape and tumor microenvironment among large cell carcinoma of the lung, large cell neuroendocrine of the lung, and small cell lung cancer. Medicine (Baltimore) 2023;102:e32781. [Crossref] [PubMed]
- Lee J, Jung YY, Lee JH, et al. The Prognostic Value of Sex-Determining Region Y-Box 2 and CD8+ Tumor-Infiltrating Lymphocytes in Limited-Stage Small-Cell Lung Cancer. Oncology 2021;99:528-38. [Crossref] [PubMed]
- Tang Y, Hu Y, Niu Y, et al. CCL5 as a Prognostic Marker for Survival and an Indicator for Immune Checkpoint Therapies in Small Cell Lung Cancer. Front Med (Lausanne) 2022;9:834725. [Crossref] [PubMed]
- Gelsomino F, Lamberti G, Parisi C, et al. The evolving landscape of immunotherapy in small-cell lung cancer: A focus on predictive biomarkers. Cancer Treat Rev 2019;79:101887. [Crossref] [PubMed]
- Liu SV, Reck M, Mansfield AS, et al. Updated Overall Survival and PD-L1 Subgroup Analysis of Patients With Extensive-Stage Small-Cell Lung Cancer Treated With Atezolizumab, Carboplatin, and Etoposide (IMpower133). J Clin Oncol 2021;39:619-30. [Crossref] [PubMed]
- Rudin CM, Awad MM, Navarro A, et al. Pembrolizumab or Placebo Plus Etoposide and Platinum as First-Line Therapy for Extensive-Stage Small-Cell Lung Cancer: Randomized, Double-Blind, Phase III KEYNOTE-604 Study. J Clin Oncol 2020;38:2369-79. [Crossref] [PubMed]
- Paz-Ares L, Garassino MC, Chen Y, et al. Durvalumab ± Tremelimumab + Platinum-Etoposide in Extensive-Stage Small Cell Lung Cancer (CASPIAN): Outcomes by PD-L1 Expression and Tissue Tumor Mutational Burden. Clin Cancer Res 2024;30:824-35. [Crossref] [PubMed]
- Goldman JW, Garassino MC, Chen Y, et al. LBA86 Durvalumab (D)±tremelimumab (T)+ platinum-etoposide (EP) in 1L ES-SCLC: Characterization of long-term clinical benefit and tumour mutational burden (TMB) in CASPIAN. Ann Oncol 2020;31:S1212-3.
- Dora D, Rivard C, Yu H, et al. Characterization of Tumor-Associated Macrophages and the Immune Microenvironment in Limited-Stage Neuroendocrine-High and -Low Small Cell Lung Cancer. Biology (Basel) 2021;10:502. [Crossref] [PubMed]
- Zhu M, Huang Y, Bender ME, et al. Evasion of Innate Immunity Contributes to Small Cell Lung Cancer Progression and Metastasis. Cancer Res 2021;81:1813-26. [Crossref] [PubMed]
- Klein S, Schulte A, Arolt C, et al. Intratumoral Abundance of M2-Macrophages is Associated With Unfavorable Prognosis and Markers of T-Cell Exhaustion in Small Cell Lung Cancer Patients. Mod Pathol 2023;36:100272. [Crossref] [PubMed]
- Kanemura H, Hayashi H, Tomida S, et al. The Tumor Immune Microenvironment and Frameshift Neoantigen Load Determine Response to PD-L1 Blockade in Extensive-Stage SCLC. JTO Clin Res Rep 2022;3:100373. [Crossref] [PubMed]
- Yang L, Zhang Z, Dong J, et al. Multi-dimensional characterization of immunological profiles in small cell lung cancer uncovers clinically relevant immune subtypes with distinct prognoses and therapeutic vulnerabilities. Pharmacol Res 2023;194:106844. [Crossref] [PubMed]
- Tian Y, Li Q, Yang Z, et al. Single-cell transcriptomic profiling reveals the tumor heterogeneity of small-cell lung cancer. Signal Transduct Target Ther 2022;7:346. [Crossref] [PubMed]
- Cai L, Liu H, Huang F, et al. Cell-autonomous immune gene expression is repressed in pulmonary neuroendocrine cells and small cell lung cancer. Commun Biol 2021;4:314. [Crossref] [PubMed]
- Shirasawa M, Yoshida T, Shiraishi K, et al. Identification of inflamed-phenotype of small cell lung cancer leading to the efficacy of anti-PD-L1 antibody and chemotherapy. Lung Cancer 2023;179:107183. [Crossref] [PubMed]
- Antonia SJ, López-Martin JA, Bendell J, et al. Nivolumab alone and nivolumab plus ipilimumab in recurrent small-cell lung cancer (CheckMate 032): a multicentre, open-label, phase 1/2 trial. Lancet Oncol 2016;17:883-95. [Crossref] [PubMed]
- Hellmann MD, Callahan MK, Awad MM, et al. Tumor Mutational Burden and Efficacy of Nivolumab Monotherapy and in Combination with Ipilimumab in Small-Cell Lung Cancer. Cancer Cell 2018;33:853-861.e4. [Crossref] [PubMed]
- Jhunjhunwala S, Hammer C, Delamarre L. Antigen presentation in cancer: insights into tumour immunogenicity and immune evasion. Nat Rev Cancer 2021;21:298-312. [Crossref] [PubMed]
- Burr ML, Sparbier CE, Chan KL, et al. An Evolutionarily Conserved Function of Polycomb Silences the MHC Class I Antigen Presentation Pathway and Enables Immune Evasion in Cancer. Cancer Cell 2019;36:385-401.e8. [Crossref] [PubMed]
- Wei Y, Zheng L, Yang X, et al. Identification of Immune Subtypes and Candidate mRNA Vaccine Antigens in Small Cell Lung Cancer. Oncologist 2023;28:e1052-64. [Crossref] [PubMed]
- Tosi A, Lorenzi M, Del Bianco P, et al. Extensive-stage small-cell lung cancer in patients receiving atezolizumab plus carboplatin-etoposide: stratification of outcome based on a composite score that combines gene expression profiling and immune characterization of microenvironment. J Immunother Cancer 2024;12:e008974. [Crossref] [PubMed]
- Gazdar A. MS32. 04 molecular phenotypes of SCLC. J Thorac Oncol 2018;13:S309.
- Dora D, Rivard C, Yu H, et al. Neuroendocrine subtypes of small cell lung cancer differ in terms of immune microenvironment and checkpoint molecule distribution. Mol Oncol 2020;14:1947-65. [Crossref] [PubMed]
- Saito M, Shiraishi K, Goto A, et al. Development of targeted therapy and immunotherapy for treatment of small cell lung cancer. Jpn J Clin Oncol 2018;48:603-8. [Crossref] [PubMed]
- Liu SV, Mok TSK, Nabet BY, et al. Clinical and molecular characterization of long-term survivors with extensive-stage small cell lung cancer treated with first-line atezolizumab plus carboplatin and etoposide. Lung Cancer 2023;186:107418. [Crossref] [PubMed]
- Xie M, Vuko M, Rodriguez-Canales J, et al. Molecular classification and biomarkers of outcome with immunotherapy in extensive-stage small-cell lung cancer: analyses of the CASPIAN phase 3 study. Mol Cancer 2024;23:115. [Crossref] [PubMed]
- Blackhall F, Jao K, Greillier L, et al. Efficacy and Safety of Rovalpituzumab Tesirine Compared With Topotecan as Second-Line Therapy in DLL3-High SCLC: Results From the Phase 3 TAHOE Study. J Thorac Oncol 2021;16:1547-58. [Crossref] [PubMed]
- Johnson ML, Zvirbule Z, Laktionov K, et al. Rovalpituzumab Tesirine as a Maintenance Therapy After First-Line Platinum-Based Chemotherapy in Patients With Extensive-Stage-SCLC: Results From the Phase 3 MERU Study. J Thorac Oncol 2021;16:1570-81. [Crossref] [PubMed]
- Paz-Ares L, Champiat S, Lai WV, et al. Tarlatamab, a First-in-Class DLL3-Targeted Bispecific T-Cell Engager, in Recurrent Small-Cell Lung Cancer: An Open-Label, Phase I Study. J Clin Oncol 2023;41:2893-903. [Crossref] [PubMed]
- Ahn MJ, Cho BC, Felip E, et al. Tarlatamab for Patients with Previously Treated Small-Cell Lung Cancer. N Engl J Med 2023;389:2063-75. [Crossref] [PubMed]
- Zhao J, Li W, Zhang X, et al. 2018P Novel nomogram based on the expression of DLL3 and PD-L1 for predicting the prognosis of small cell lung cancer patients. Ann Oncol 2023;34:S1062-S1079.
- Lakhani NJ, Rasco DW, Zeng Q, et al. First-in-human study of palcitoclax (APG-1252), a novel dual Bcl-2/Bcl-xL inhibitor, demonstrated advantages in platelet safety while maintaining anticancer effect in US patients with metastatic solid tumors. J Clin Oncol 2020;30:3509.
- Mita A, Loeffler M, Bui N, et al. A phase I study of tinostamustine in patients (pts) with advanced solid tumours. Ann Oncol 2019;30:v177-8.
- Pietanza MC, Litvak AM, Varghese AM, et al. A phase I trial of the Hedgehog inhibitor, sonidegib (LDE225), in combination with etoposide and cisplatin for the initial treatment of extensive stage small cell lung cancer. Lung Cancer 2016;99:23-30. [Crossref] [PubMed]
- Owonikoko TK, Niu H, Nackaerts K, et al. Randomized Phase II Study of Paclitaxel plus Alisertib versus Paclitaxel plus Placebo as Second-Line Therapy for SCLC: Primary and Correlative Biomarker Analyses. J Thorac Oncol 2020;15:274-87. [Crossref] [PubMed]
- Ellis PM, Shepherd FA, Laurie SA, et al. NCIC CTG IND.190 phase I trial of dalotuzumab (MK-0646) in combination with cisplatin and etoposide in extensive-stage small-cell lung cancer. J Thorac Oncol 2014;9:410-3. [Crossref] [PubMed]
- Pietanza MC, Waqar SN, Krug LM, et al. Randomized, Double-Blind, Phase II Study of Temozolomide in Combination With Either Veliparib or Placebo in Patients With Relapsed-Sensitive or Refractory Small-Cell Lung Cancer. J Clin Oncol 2018;36:2386-94.
- Farago AF, Yeap BY, Stanzione M, et al. Combination Olaparib and Temozolomide in Relapsed Small-Cell Lung Cancer. Cancer Discov 2019;9:1372-87. [Crossref] [PubMed]
- Byers LA, Bentsion D, Gans S, et al. Veliparib in Combination with Carboplatin and Etoposide in Patients with Treatment-Naïve Extensive-Stage Small Cell Lung Cancer: A Phase 2 Randomized Study. Clin Cancer Res 2021;27:3884-95. [Crossref] [PubMed]